Introduction
Soft, porous crystals are porous solids that pass a highly ordered network and structural transformability (Sugiyama et al, 2012). Soft or flexible porous metal-organic frameworks (MOFs) have found applications in molecular recognition, selective gas adsorption/separation, or chemical sensing (Tan &cheetham, 2011). When compared with solid porous MOFs, the soft, porous crystal contains pronounced structure flexibility when exposed o an external inducement like gas molecules, pressure, and temperature (Tan et al, 2012). It is a unique property of SPCs. The structure of soft, porous crystals responds very sensitively to small external inducement or stimulus that presents a challenge to molecular dynamic simulations with flexible size and shape of the simulation cell. Thus, to obtain the right equilibrium structures, all the interactions must be precise.
Metal-organic frameworks (M OFs) are an interesting class of porous solids. There is an increasing number of MOF s with different pore shapes, sizes, and structure compositions that are still under research in recent years. Most MOFs are stable have solid structures but there exists a class of MOFs with flexibility in their structure. It means that they have a unique characteristic forming the basis of soft, porous crystal discovery. According to Kitagawa et, al.3 has argued that the way host molecules react with guest molecules causes gate opening.
Influencing gas sorption properties
Gas adsorption properties of solid MOFs can be enhanced by functionalizing their organic groups. But the same approach to flexible MOFs has not been widely discussed or researched. The functionalization process causes the physical and chemical properties of solid MOFs structures, to be modified. Some of the properties include change of polarity, acidity, basicity, and host-guest molecule interaction.
The above modification has a positive impact on gate opening properties and the adsorption capacity of MOFs. From recent research, it was discovered that the availability of functional groups influences the initial breathing phenomenon and the selective adsorption of liquid guests because of individual guest framework interactions. Despite the exceptional highly flexible phenomenon, there is little research concerning this family partly because of its complex structure.
The single porous crystals have a 3-dimensional framework that forms metal centers joined by organic ligands presenting significant structural varieties and chemical versatility that enable new materials with tenable host-guest properties to be designed (Tan &Cheetham, 2011). Single porous crystals under some external physical constraints that include temperature, guest adsorption, and mechanical pressure exhibit reversible single-crystal-to-single-crystal structural transformation of substantial amplitude. According to material scientists and engineers, some of the practical applications include nanotechnology, sensors for organic molecular detection, and precise gas separation (Ortiz et al, 2012).
This research review explores the properties of these materials while addressing the interplay between the phenomenon of absorption, deformation, and the reaction of these materials. In addition, the research shows the link between the structure and properties of soft, porous crystals (Tan et al, 2012). Different chemistry techniques have been used to understand the behavior of metal-organic frameworks and, in particular, single porous crystals. First, is the use of macroscopic thermodynamic models which utilize experimental data and theoretical calculations, the second technique is static quantum chemistry calculations which give more understanding of the microscopic scale on host properties and host-guest interactions. Third, is the use of force field molecular simulation methods and, in particular, Molecular Dynamics (MD) and Monte Carlo (MC) techniques? These methods examine the phase space of the system being studied (Tan &Cheetham, 2011
Framework of Mofs
The flexibility of frameworks of the MOFs
The flexibility of MOFs is a result of two factors which are: the nature of the crystalline network arrangement and combined framework transition. MOFs materials have a unique property, which does not have rigid state materials and responds to both physical and chemical inducement. MOFs can be tuned using the molecular design of the framework. The transformation of phases is through adsorption or desorption of guest molecules, photochemical, temperature, and physical stimuli. The flexible characteristics of MOFs, e.g., linker rotation, and sub-network sliding are present in this research work. These properties are not affected by the crystallographic phase transformation. Flexible MOF systems discussed in this research review can be classified according to the type of metal joints involved. The classification is also based on how this network harmonizes with the organic component molecules to constrain the framework dynamics. Also, the harmonization of the flexible and responsive characteristics through the concept of solid-solution mixture is covered
Breathing
Kitagawa 1998 anticipated that MOFs can be flexible when he put the porous coordination polymer into three generations: first, second, and third generations. The breathing phenomenon observed in 2D layered frameworks confirmed Kitagawa’s prediction.
Soft, porous crystal represents a particular subclass of MOFs with remarkable flexibility. They display a vast spectrum of flexibility mechanisms. It includes loaded structure dynamics such as adsorption-induced linker representation in ZIF-8 (Ortiz et al, 2013). Also, negative thermal expansion as in the IRMOF family of materials, gradual swelling upon uptake of solvent molecules as in the MIL-88 family, and gate opening. Gate opening involves an abrupt structural transition between closed and open-pore structures. It is induced by gas adsorption as in the ELM family and the breathing phenomenon as in the MIL-53 family (Ortiz et al, 2013). The synthesis of MOFs by introducing flexibility constituents on the BDC-type linker exhibited the breathing phenomenon that was explained independently by Cohen and Fischer (Cohen, 2010). Some soft, porous MOFs are represented only in 2D layer frameworks that can cause layer slides when dissolved. Also, a 3D porous structure can experience the reversible structural transition between narrow pore (np) and large pore (LP) phases.
One of the setbacks of MOFs is the low thermal stability, but they have a flexible structure that yields to gate opening effect. The breathing phenomenon characteristics of the MOFs enable scientists and engineers to use them in various fields such as sensing, gas storage, and separation (Llewellyn et al, 2006). But some classes of MOFs tend to exhibit the breathing phenomenon. For instance, MO4 (OH) 2- octahedral and 1, 4-benzene dicarboxylic (BDC) acids are used to construct the MIL-53 series. The small pore structure has a hydrogen bond interaction between the water molecules and hydrogen atoms (Mays et al, 2014). At elevated temperatures, dehydration occurs on the MIL-53s causing the absence of the hydrogen bond interactions between hydrogen and water molecules. It results in MIL-53 exhibiting a porous structure as a large pore.
Fig. 1 shows how the breathing phenomenon in MIL-53 by subjecting it to heat. (a) Dehydrated LP form; (b) hydrated np form. Hydrated MIL-53 expresses revolutionary adsorption behavior when exposed to CO2 molecules. The hydrated MIL-53 changes in the following stages: large pore mixture of a large pore and narrow pore –pure large pore depending on a pressure range of CO2 molecules. The CO2 pressure of 5 bars and below makes the hydrated MIL-53 exist in its small pore form. The framework has large pore and small pore forms which exist together harmoniously as the pressure is increased. When the gas pressure is, the structure transforms into a pure large pore form (Li et al, 2009).
For dehydrated MIL-53, the breathing behavior is different. They exhibit a two-step structure transition upon CO2 adsorption, i.e., LP-np-LP. The first stage takes place at approximately 3mm/g while the second stage takes place at around 8 bars. It occurs in the presence of water molecules immediately before the adsorption, causing a steric barrier and hence the occurrence of the final stage of the structural transformation. The hydrated MIL-53 shows small CH4 uptake when it is close to the dehydrated one. This behavior has broad application in gas storage and separation e.g selective adsorption of CO2 over CH4 (Murray et al, 2009, Klein et al, 2012). The reaction between the guest molecules and the host framework causes the effect of the gate opening in MOFs. To understand the breathing dynamics powder X-ray diffraction is used to display the structural transformation that interferes with the gas adsorption capability (Neimark et al, 2011).
CO2 adsorption and mechanical compression mechanisms of framework transformations are within a unified thermal model depending on the reduction of the osmotic potential. The pressure induced on the inside of the guest molecule’s pores is a function of the size of the external stress of mercury applied.
When the osmotic ensemble performs a thermodynamic analysis of equilibrium states, the structural transformation can be described by a general framework that is demonstrated by the adsorption stress experiment when achieving the threshold stress is made. The transformation conditions and their respective phase diagrams at equilibrium can form single cells using different simulation approaches (Bousquet et al, 2013). However, this thermodynamic treatment does not yield any result in the local elastic deformity of the material nor on the alleyways and dynamics of structural transformations.
Early research and studies put more emphasis on fundamental scalar properties that include bulk modulus and some of them specific to key crystallographic axes like Young’s modulus, hardness (Remy et al, 2011). Recently, it has been demonstrated that, in the case of ZIF-8, the experiments are carried out using advanced pieces of equipment adequately accounting for the anisotropy of framework elastic characteristics (Ravikovitch&Neimark, 2006). Based on either quantum chemistry calculations or classical molecular dynamics, the tensor analysis of elastic properties shows a great deal of information about flexible MOFs. Using ab initio calculations of the elastic reveals that high anisotropy and different directions of a low Young and shear module are the key signatures of “breathing or gate opening effect” MOFs (Coudert et al, 2008). For instance, those of a wine-rack framework topology, such as the MIL-53 family (Boutin et al, 2009).
The anisotropy can achieve a 400:1 ratio between the most rigid and weakest direction when comparing the properties of 3D maps of an elastic module for MIL-47 and MOF-5 structures. The ratio of the elastic module in “soft” and “hard” directions is approximately 100 for flexible MIL-47.
Fig.2Comparison of the directional Young’s modulus of breathing MOF MIL-47 and non-breathing MOF-5.
The large pore (LP) to narrow pore (np) structural transition in MIL-53 can be generated either by mechanical pressure or adsorption. It is systemized in the lower half of the graphics. (Top left) Intrusion-extrusion experiments in mercury, attributed to the difference in the volume of the sample (Δ V) as a function of mechanical pressure. The two clear stages are seen corresponding to large pore−narrow pore and narrow pore –large pore transitions. Adsorption and desorption isotherms of CO2 at 304 K. High anisotropy and anomalously low Young and shear module are the key signatures of ” breathing” MOFs. It is only ∼ 2 for noncompliant MOF-5. In addition, it is proved that the application of hydrostatic pressure on ZIF-8 leads to a shear weakening and subsequent mechanical breakdown that may provoke the crystal to become amorphous (Coudert, 2010). While analyzing the adsorption-induced deformation, an important factor that should be considered is the influence of the adsorbate of the crystal elastic module, which was earlier demonstrated by Coasne et al..
Swelling of MIL-88 family
The characteristic of the space group, MIL-88 type framework, was investigated by carrying out synchrotron x-ray powder diffraction tests. It was achieved by using glass capillaries filled with the title powders disposed of at room temperature in polar liquids. Modified MIL-88 materials and non-modified MIL-88B and D solids exhibit similar cell parameters. Even though, there are small changes in the unit cell volume increase in the open modified MIL-88 solids with a unit cell volume increase to 100A3 when compared to the non-modified stable. It is because of the availability of substantial functional groups that need the structure to expand to a bigger dimension so that it can encompass the guest molecules.
The existence of strong hydrogen bonding between the hydroxyl groups of the linker and of the alcohol molecules strengthens a partial pore opening. It is during adsorption that one could also point out that the MIL-88B(2OH) solid presents a transitional unit cell volume of 2800 Å3 in ethanol vs. 32003500 Å3 for the other analogs. The crystal structures of the modified MIL-88B and MIL-88D Solids in both dried and open states. The states have been determined using a computationally assisted structure determination strategy. The strategy is based on energy minimization procedures and classical force fields for representing the interactions within the framework. The results showed that indeed, all the functionalized structures were initially constructed from the initial atomic coordinates of the non-modified MIL-88B and MIL-88D frameworks. The results also indicated that further geometry was optimized by enforcing the experimental unit cell parameters acquired from the X-ray diffraction refinement journal how linkers modification (Neimark et al, 2011). Using the argument based on the simulated structures, the origin of the decrease in the swelling magnitude can be explained in terms of steric hindrance. The MIL- 88 structures when in the dried forms contract along with the a and b directions while expanding in c directions (Patricia et al, 2011). It results in an overall decrease in unit cell volume. The opening of the structures has an angle (θ) formed between the linker and the bipyramid height. The dried MIL-88B structure interacts through π-π interaction since the phenyl rings are located either above or below the trimmers, almost packed along the c axis.
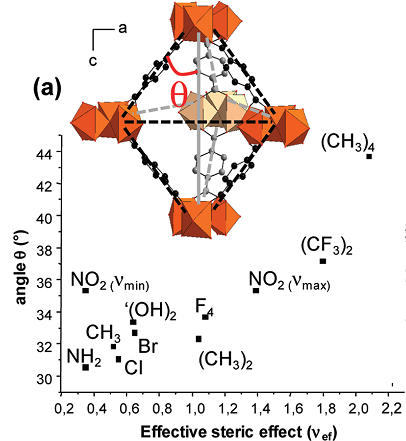
This makes the structure be stabilized in its closed form.
Swelling as a Function of the Solvent Properties
The longer the organic space in MIL-88, the harder is the pore opening in the liquid state. For instance, the dried MIL-88D required the use of hot pyridine to reopen its pores whereas the partially opened MIL-88D in its as-synthesized form can be fully re-established in some selected liquids. A lighter π-stacking effect can explain this in the partially open MIL- 88D structures, due to the possible rotation of the two phenyl rings, as it can be deduced from the simulated structures (Schneemann et al, 2014). The attribute is also following the easier accessibility of the porosity, starting from a partial pore opening as observed for the N2 adsorption on partially activated MIL-88 samples (Savonnet et al, 2010).
Functionalized linker
Two different methodologies can be applied for the implementation of additional functionalities within MOFs. These include functionalization of the linker before the MOFs synthesis by synthetic organic chemistry. Also, the modifying the linker after the construction of the framework through a chemical reaction of already implemented functional groups of the linker using suitable reactants.
The pore size affects the adsorptions rate. It also affects the gas uptake and gas adsorption rate. To manufacture new MOFs one requires new function linkers with different sizes and shapes. This method has the following advantages:
- It enables the pore size to be changed according to the requirement.
- Adsorption rate is substantially increased.
- The separation power of MOFs is enhanced.
Functionalized linker ligands are very unstable, reactive, and have high solubility under the normal reaction conditions. It makes the inclusion of pre-functionalized linkers to be uniform. This challenge has prompted more research on MOFs framework modification of its functional groups using PSM (Cohen, 2010, Wang & Cohen, 2009). PSMs cannot be implemented without the following conditions: admission of MOF porosity, functional linker being present, and the conditions under which the reaction takes place are stable. (Serre et al, 2007). In PSM, the reaction conditions for the MOFs synthesis are known. It enables a modification of a particular MOF to attain an iso reticular functionalized network.
MIL-53 experiences a small expansion of unit cell volume when amine organic linkers are included in the structure. Even so, the above behavior does not bring any change in the structure of MOFs nor the breathing practices. Due to the existence of amine groups in functionalized MIL-53 in the inside of its channels, it results in the expansion of unit cell volume, less adsorption capacity, and thermal stability of the structure.
When NH2-MIL-53 was examined, the same effect was observed with a considerable reduction of CO2 adsorption that is characterized by less available micropores volume. The ability of CO2 to be separated by the NH2-MIL-53 series is higher when compares to CH4. It is determined by the reaction between the MIL-53 series structure and the individual molecules of NH2 function groups and CO2 molecules (Ibara et al, 2010. Various MIL-53 can be constructed using PSMs. From the investigation carried out on the MOFs’ breathing behavior, it was confirmed that the behavior is different depending on the function linker attached to it (Yoon et al, 2007).
The transition of MOFs from large pore to narrow pore and vice versa can be attributed to the hydrogen bonding formed during the transition cycle between the adsorbents and absorbents. However, in some cases, it is observed that the extraction of water molecules initiates the transition cycle but at a higher temperature.
The framework of F-MIL-53(Al) is very rigid which probably results in decreased n-hexane adsorption capacity. Functionalized MIL-53s, for example, that of AL’s, exhibited slightly smaller accessible micro pores volumes compared with their parent material. It is due to the occupancy of functional groups inside the one-dimensional channels. The available micro pores volumes demonstrate the decreasing trend due to the steric hindrance effect of functional groups. This fact affects the adsorption capacities of CO2 molecules too.
Interpenetration control and related functionalized applications
The use of long organic components to design the frameworks frequently results in interpenetrated MOFs with smaller pores. The interpenetration process often makes MOFs robust. Although, it harms the porosity of open structures with the reduction of open-pore size. Once the frameworks of the MOFs are interpenetrated extensively, the final structure contains low porosity, small surface area, and high density. This phenomenon affects negatively, the potential applications of such materials since high surface area and porosity are the most desired characteristics in porous materials. For instance, when MOF-5 was subjected to several examinations in the past several years, it reported revealing adsorption properties with noticeable differences. The report displayed specific surface areas in the range from 570 to 3800 m2/g and H2 uptakes from 1.3 to 7%. The period of MOF-5 exposure to air-water was found to be an essential point to its phase purity and gas sorption capacities as discovered by the Long group (Bloch et al, 2010). Immediately after that, the ligand group used the single-crystal XRD to conduct more research on a structural contrast between the MOF-5 with high and low surface areas. The MOF-5 sample with a small surface area had two different types of crystals. One of the phases had less gas adsorption capacity and a relatively smaller surface area than the anticipated values because it composed 2-fold interpenetrated MOF-5 networks. Dissimilarly, the cavities in the other phase were partially replaced with Zn(OH)2 species. It inevitably makes the hosting cavity, and possibly also adjacent holes, inaccessible, and thus, this reduces the pore volume of the material. Hence, it is crucial to come up with strategies to remove the interpenetration from the frameworks to come up with highly porous MOFs that have high surface area. The Yaghi group introduced the use of infinite SBUs to address this issue. They successfully built 4, 4- Biphenyl dicarboxylate (bpdc, Scheme 1)-based Zn-MOFs were bearing Zn O C columnar units that run along one direction. The topology of the framework of these MOFs is the same as that of the Al net in SrAl2. However, all these MOFs contain an open structure in which Zn O links within the SBUs when they are compared to original SrAl2 and C6H4 C6H4 links between the SBUs, expanding the Al net. The inherent order of these rods in the framework prevents interpenetration. Subsequently, they highlighted the importance of the concept regarding the rod SBUs when designing and preparation of a series of MOFs. Such methodology may be applied to organic components (linker) than bpdc. However, they must have the same width (one benzene ring) and could be used in similar syntheses to come up with the same framework but with larger pores accordingly. Moreover, the controllable construction of rod SBUs is too difficult to analyze, and it is associated with many factors concerning the final MOF structures. Framework interpenetration control in MOFs has continued to be researched by various groups who have dedicated their work to regulating the synthetic variables or approaches in the last decade.
The reaction temperature and concentration control in MOFs
Some of the important reaction parameters that have been found in controlling the framework interpenetration of MOFs are temperature and concentration. It has been found that longer Organic linkers readily lead to 2-fold interpenetrating structures during their synthesis of the iso reticular MOF series. Nonetheless, the more the reaction solutions are diluted; the synthesis of non-interpenetrating MOFs with larger pores takes place. With the help of this research, pairs of isomers for iso reticular MOF-0, -12, -14, and -16 were obtained. And of importance is that each pair is composed of one on interpenetrated. The other is composed of 2-fold interpenetrated frameworks. The variation of temperature and concentration for controllable syntheses of a non-interpenetrated form of [Cd(4,4-bpy)( BDC)]•3DMF•H2O (compound 1) and its previously reported 2-fol d interpenetrated form, have been systematically researched. It was evident that the non-interpenetrated can be precisely obtained by reacting Cd ( NO3)2•4H2O,4,4-bpy, and H2bdc in a 1:1:1 molar ratio in DMF/DEF (2:1, v/v) at85 ◦C. Compound 1 contains 4,4-bpy as pillars link a 3D network with a pillar-like layer structure. The layers are composed of Cd2N4O8 clusters and benzene carboxylic ligand. The resultant3D framework has 8.1 A˚ × 11.7 A˚ square channels along the a-axis and 12.5 A˚ × 12.5 A˚ passages in rhombic structure along the c-axis that are occupied by disordered DMF and H2O solvents. The non-interpenetrated structure of 1 is surprisingly similar to the previously reported single net of the double interpenetrated compound 2. To rationalize the effect of reaction state for the framework interpenetration, the authors carefully adjusted the temperature and concentration parameters, and the results clearly showed that both variables subtly affected the interpenetration and product purity. Based on these studies, they concluded that the interpenetrated isomer was preferentially produced at elevated temperatures. Also, lowering the concentration of starting materials in a particular range reduced the possibility of forming a sub-lattice in the cavities of a non-interpenetrated framework. Recently, based on the same reaction starting materials, non-porous to microporous MOFs have since been synthesized. The synthesis uses the same reaction materials from the start of the process through interpenetration control. It is achieved by reducing the temperature of the reaction. Micro pores have been further enlarged to mesopores by only decreasing reactant concentrations and reducing response time ligand design /modification-induced control.
Another approach to efficiently prevent the interpenetration of MOFs framework is the presence of the steric bulkiness of groups on the linker ligand. Consequently, the above objective can be realized by selective modification of a ligand with medallion groups. The structural interpenetration can be effectively prevented by first introducing the bulky constituents on the BDC ligand, but the framework structure remains fixed to be (6, 3) net topology (Zou et al, 2010). Interpenetration has been suppressed by pure ligand design and succeeded in the preparation of a series of interpenetration framework isomers (Kim et al, 2010). All MOFs obtained from L6 are non-Interpenetrated whereas 2-fold interpenetrated structures are obtained with L5 under similar reaction conditions. The main difference between L5 and L6 is the change from aryl-H to aryl-Br moieties carrying on the tetra-topic carboxylate ligand, which according to the experiments carried out, has been demonstrated to be efficient for interpenetration prevention.
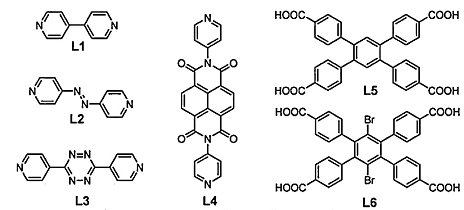
The experiments carried out concerning the CO2 sorption show that there exist differences in porosity and sorption properties between interpenetrated 10 and non-interpenetrated 11. Hence, the potential dynamic structural phenomenon in interpenetrated 16, gives rise to the steps in the isotherm at P/P0 approximately 0.022 when it is activated and guest adsorption. It occurs when the behavior disappears in 17 with a non-interpenetrated network. All these results demonstrate that interpenetration is a phenomenon that plays a significant role in the framework dynamics. In addition, a gas sorption property in MOFs in return has a direct impact on the swelling, linker rotation, and breathing characteristics of MOFs.
Subsequently, with standard biphenyl dicarboxylate linkers with one or two pendant azolium moieties, two cubic Zn-based MOFs have been obtained with interpenetrated and non-interpenetrated structures, respectively. Results have indicated that the introduction of different numbers of bulky azolium moieties (one vs. two) onto the linear dicarboxylate linkers can control Structural interpenetration in MOFs [59b]. Reactions of BDC, 4, 4-bpy and cobalt (II) salt in DMF gave a nonporous, doubly interpenetrated MOF, Co2 (BDC) 2(4, 4-bpy) 2. In contrast, similar reaction conditions using 2-amino-1,4- benzene dicarboxylate realized the formation of a porous layer of MOF that is double pillared. It is without interpenetration, [Co2(and)2(4,4-bpy)2]•8DMF, with permanent porosity. The results have further demonstrated that the introduction of bulky substituents on the organic linkers could be a general method for interpenetration control ( Liu et al, 2010). Four structurally similar rod-like ligands (Scheme 1 ), 1,4-bis(benzimidazole-1-yl)-phenyl, 1,4-bis(imidazol-1-yl)-benzene,4,4-bis(imidazol-1-yl)-biphenyl, and 4,4-bis(benzimidazole-1-yl)biphenyl have been employed to react with Co(II) or Cd(II) salts. They produce 3D MOFs with different degrees of interpenetration under similar conditions. Structural analyzes confirmed that both coordination mode and spacer length play significant roles in the determination of the degree of interpenetration of MOFs, which also could be tenable by ligand modification, such as varying the ligand space or terminal group.
The successful syntheses of not interpenetrated and interpenetrated Cu-MOFs with the expanded sodalite-type network by respectively employing benzene- and triazine-centered versions of an elongated triangular N-donor ligand have been reported. It is concluded that the reason for the interpenetration manipulation for the structures is not only because of the three different atoms (C or N) involved in the two ligands. It is also mainly due to the presence of some degree of torsion between the central, and outer phenyl rings in the benzene-centered ligand. However, such strain does not exist in the triazine-centered one. It is found that the non-interpenetrated structure, although it affords larger pore space, is prone to collapse upon desolvation. However, interpenetration can stabilize the other framework, leading to increases in both surface area and hydrogen storage capacity. The result indicates that interpenetration control is an important factor in the synthesis of hydrogen storage materials. Most recently, interpenetration control in MOFs with similar net topologies can be manipulated via a simple change in the ligand by replacing a C-C bond with a C-C bond.
Application of Flexible Framework
The concepts highlighted, and the methods described in this research review are not limited to SPCs but can also be applied to other compliant porous solids. The phenomena of adsorption deformation and structural transitions in SPCs have attracted a lot of recent interest because of the large-scale stimuli-induced deformations. Some of the areas, where thermodynamic and mechanical models could be of significant use include deformation of micro-and mesoporous adsorbents and catalysts under technological conditions. Also, the swelling of clays and laminated minerals, shrinkage, and swelling of coal and its influence on the secondary methane recovery and carbon dioxide sequestration. In addition, mechanical properties of low- k dielectric films, hydrofracturing in shale gas reservoirs, hydration-dependent mechanical properties of cement and other building materials, porous membranes, and electrodes in fuel cells and batteries. Although some attempts in these directions have been already made, a comprehensive poromechanics of nanostructure adsorbents remains to be built (Francois et al, 2013).
CO2 adsorption in MOFs
Reducing the CO2 levels in the atmosphere is one of the most challenging phenomena for modern human society. From the recent research on the effects of the CO2 gas, it is partly responsible for climate change (Sarah, 2012).
Series of these MOFs with different features; framework flexibility, topology, and functional groups are screened to determine how much they can absorb CO2 and CH4. Following the screening, one specific material, amino-MIL-53, a framework with amine groups attached to its pore wall, was substantiated to be very discerning.
Like most porous materials, CO2 adsorption and uptake in MOFs are dependent upon the size/volume of the pore and surface dependency. CO2 adsorption isotherm in a rigid porous material, such as traditional zeolites and the majority of rigorous MOFs, presents a regular type-I shape, but in a few stiff MOFs, stepwise isotherms have been observed. It can be related to the structural features of these MOFs, e.g..Zhang et al. reported the two-step CO2 adsorption at low temperature in a highly connected MOF, NiII2NiIII(3 -OH) (PBA)3 (2,6-ndc)1.5 (MCF-19; PBA = 4-(pyridin-4-yl)Benz(…) contains a two-way porous framework with cavities connected to each other. Pore MOFs, like o IRMOF-1, BDC = 1,4-benzenedicarboxylate), Zn4O(btb)2 (MOF-177,btb = benzene-1(…)(benzene-1,3,5-triyltris(ethyne-2,1- di-yl tri-benzoate , BPDC = biphenyl-4,4-dicarboxylate) at close to 250c and high pressure. In these cases, this behavior is experienced because of its admirable electrostatic interactions between CO2 molecules which cause significant liquefaction, which has been explained by which demonstrated Snurr and co-workers, in terms of molecular simulation. But flexible MOFs contain a gradual absorption and show hysteretic desorption isotherms for CO2 and other gaseous compounds. This characteristic makes MOFs different since they demonstrate high potential in particular adsorption and separation of gaseous compounds (Yazaydim et al, 2009).
Some of the MOFs that show the breathing phenomenon is the MIL-53 during the adsorption and desorption processes of CO2. This phenomenon has been linked to the phase transition of MOF frameworks. For instance, the phase shift in MIL-53(Cr) can be demonstrated using the X-ray diffraction method that follows the gas adsorption path. Similarly, CO2 adsorption in Sc2( BDC- three ) at 235 K and 1 bar resulted in an asymmetry change of crystal structure from orthorhombic F-DDD to monoclinic C2/c through the tilting of BDC linkers. CO2 molecules take up different adsorption sites in two symmetrically, different channels of the framework that result from this symmetry change. A post-synthetic modification strategy has also) been used to modulate the breathing behavior of MOFs. Cohen and co-workers reported the change of DMOF-1-NH2, Zn2 (NH2-bdc) 2(dabco) (dabco = 1, 4-diazabicyclo [2.2.2] octane), toN (…) where ‘n’ denotes the chain length of the newly formed linear alkyl amide. Systematic CO2 adsorption measurements on DMOF-1-AM(n – 1) showed that the breathing is related to the alkyl chain length of the post- added groups. The step adsorption and hysteresis of desorption a196 K for DMOF-1-AM3 are very similar to that observed in MIL-53. Another example, Ni( bpee )Ni(CN)4 ( bpee = trans-1,2-bis(4 pyridyl)ethylene), reported by Culp and coworkers, is a three-dimensional (3D) work constructed by a flexible organic bridging ligand, bpee, pillaring two-dimensional (2D) rigid (Xiang et al, 2011).
The impact on CO2 adsorption in MOFs by various other factors has also been explored. Kajiro and coworkers examined the effect of metal ions on the CO2 adsorption in two iso-structural MOFs, M (4, 4-bipy) 2(OTf) 2 (M = Cu and Co). Shrinkage or adjustments of these MOF structures are largely dependent on the metal ions. As an alternative strategy, partial doping of MOF-5with Co (II) by isomorphic substitution during synthesis has been investigated to explore the effect on CO2 adsorption (Kim et al, 2010).
Conclusion
Framework interpenetration and interpenetration control have recently become topics of interest in MOF for researchers. In this research review, the primary focus has been on the interpenetration of structures built upon rigid linkers. But still, it is also observed most of the time that MOFs with flexible linker ligands presents diverse and fantastic structures. In this review, the difference between interpenetration and interweaving was not demonstrated but in detail, it exists. The term interweaving tends to be used when the interlocking of multiple networks enhances the stability of a single system but blocks potential adsorptive sites. Interpenetration is more often used when the pore size is decreased without blockage o adsorptive sites, thus maximizing the exposed surfaces of each network. We have summarized several routes reported to realize interpenetration control in MOFs. Reaction temperature or concentration control, template-directed control, ligand design/ modification-induced control, coordinated or uncoordinated solvent removal/addition-triggered control and layer-by-layer assembly. So far, it is still hard to conclude some general rules to control framework interpenetration because numerous aspects can impact the formation of the final structures of MOFs. While the first three strategies have been demonstrated to work well with different research groups or in various MOFs, no method has been identified that may universally apply. Although, it seems the layer-by-layer approach should be able to serve as interpenetration control in quite a variety of cases. However, it would be relevant only for small-scale MOF fabrication. These reported routes and strategies may be used or modified for future efforts to control framework interpenetration. Generally speaking, interpenetration can be used for practical advantage if one desires a MOF with small pore sizes, which could be beneficial to H2 and CO2 uptakes. Groups can be substituted into the MOF framework through chemical treatment.
References
A. Demessence, D.M. D’Alessandro, M.L. Foo, J.R. Long, J. Am. Chem. Soc. 131(2009) 8784.
A. Schneemann a, V. Bon b, I. Schwedler a, I. Senkovska b, S. Kaskel *b and R. A. Fischer aAnorganische Chemie II – Organometallics and Materials, Ruhr-Universität Bochum, Universitätsstr. 150, D-44801 Bochum, Germany. E-mail: [email protected] Department of Inorganic Chemistry, Technische Universität Dresden, Bergstr.66, D-01069.
Bloch E.D, D. Britt, C. Lee, C.J. Doonan, F.J. Uribe-Romo, H. Furukawa, J.R. Long, O.M. Yaghi, J. Am. Chem. Soc. 132 (2010) 14382.
Bousquet, D.; Coudert, F.-X.; Fossati, A. G. J.; Neimark, A. V.; Fuchs, A. H.; Boutin, A. Adsorption Induced Transitions in Soft Porous Crystals: An Osmotic Potential Approach to Multi stability and Intermediate Structures. J. Chem. Phys. 2013, 138, 174706.
Boutin, A.; Springuel-Huet, M.-A.; Nossov, A.; Gede ́ on, A.; ́Loiseau, T.; Volkringer, C.; Ferey, G.; Coudert, F.-X.; Fuchs, A. H. ́Breathing Transitions in MIL-53(Al) Metal −Organic Framework upon Xenon Adsorption. Angew. Chem., Int. Ed. 2009, 48, 8314−8317.
Coasne, B.; Haines, J.; Levelut, C.; Cambon, O.; Santoro, M.; Gorelli, F.; Garbarino, G. Enhanced Mechanical Strength of Zeolites by Adsorption of Guest Molecules. Phys. Chem. Chem. Phys. 2011, 13, 20096 −20099
Cohen, S.M. Modifying MOFs: New chemistry, new materials. Chem. Sci. 2010, 1, 32–36.
Coudert, F.-X. The Osmotic Framework Adsorbed Solution Theory: Predicting Mixture Co adsorption in Flexible Nanoporous Materials. Phys. Chem. Chem. Phys. 2010, 12, 10904 −10913.
Coudert, F.-X.; Jeffroy, M.; Fuchs, A. H.; Boutin, A.; MellotDraznieks, C. Thermodynamics of Guest-Induced Structural Transitions in Hybrid Organic-Inorganic Frameworks. J. Am. Chem.Soc.2008, 130, 14294 −14302.
Francois-Xavier Coudert, ̧ *, Anne Boutin, Alain H. Fuchs, and Alexander V. Neimark, Adsorption Deformation and Structural Transitions in Metal-Organic Frameworks: From the Unit Cell to the Crystal Journal of physical chemistry letters, sept.2013.
Ibarra, I. A.; Lin, X.; Yang, S.; Blake, A. J.; Walker, G. S.; Barnett,S. A.; Allan, D. R.; Champness, N. R.; Hubberstey, P.; Schr€oder, M. Chem. Eur. J. 2010, 16 (46), 13671.
Kim k. , M. Banerjee, M. Yoon, S. Das, in: M. Schröder (Ed.), Functional Metal-Organic Frameworks: Gas Storage, Separation and Catalysis, Springer, Berlin/Heidelberg, 2010, p. 115
Klein, N.; Hoffmann, H.C.; Cadiau, A.; Getzschmann, J.; Lohe, M.R.; Paasch, S.; Heydenreich, T.; Adil, K.; Senkovska, I.; Brunner, E.; et al. Structural flexibility and intrinsic dynamics in the M2(2,6-ndc)2(dabco) (M = Ni, Cu, Co, Zn) metal-organic frameworks J. Mater. Chem. 2012, 22, 10303–10312.
Li, J.; Kuppler, R.J.; Zhou, H.-C. Selective gas adsorption and separation in metal-organic frameworks. Chem. Soc. Rev. 2009, 38, 1477–1504.
Liu Y, W. Xuan, Y. Cui, Adv. Mater. 22 (2010) 4112.
Llewellyn, P.L.; Bourrelly, S.; Serre, C.; Filinchuk, Y.; Férey, G. How hydration drastically improves adsorption selectivity for CO2 over CH4 in the flexible chromium terephthalate MIL-53. Angew. Chem. Int. Ed. 2006, 45, 7751–7754.
Loiseau, T.; Serre, C.; Huguenard, C.; Fink, G.; Taulelle, F.; Henry, M.; Bataille, T.; Férey, G. A rationale for the large breathing of the porous aluminum terephthalate (MIL-53) upon hydration. Chem. Eur. J. 2004, 10, 1373–1382.
Mays Alhamami; Huu Doan; Chil-Hung Cheng, A Review on Breathing Behaviours of Metal-Organic-Frameworks (MOFs) for Gas Adsorption.
Murray, L.J.; Dincă, M.; Long, J.R. Hydrogen storage in metal-organic frameworks. Chem. Soc.Rev. 2009, 38, 1294–1315.
Neimark, A. V.; Coudert, F.-X.; Triguero, C.; Boutin, A.; Fuchs,A. H.; Beurroies, I.; Denoyel, R. Structural Transitions in MIL-53 (Cr): View from Outside and Inside. Langmuir 2011, 27, 4734 −4741.
Ortiz, A. U.; Boutin, A.; Fuchs, A. H.; Coudert, F.-X. Anisotropic Elastic Properties of Flexible Metal-Organic Frameworks: How Soft Are Soft Porous Crystals? Phys. Rev. Lett. 2012, 109, 195502.
Ortiz, A. U.; Boutin, A.; Fuchs, A. H.; Coudert, F.-X. Investigating the Pressure-Induced Amorphization of Zeolitic Imidazolate Framework ZIF-8: Mechanical Instability Due to Shear Mode Softening. J. Phys Chem. Lett. 2013, 4, 1861 −1865.
Ortiz, A. U.; Boutin, A.; Fuchs, A. H.; Coudert, F.-X. Metal-Organic Frameworks with Wine-Rack Motif: What Determines Their Flexibility and Elastic Properties? J. Chem. Phys. 2013, 138, 174703.
Patricia H.,Fabrice S., Stefan W.,Thomas D.,Daniela H.,Guillaume M., Alexandre V.,Marco D.,Olivier D.,Emmanuel M., Norbert S., Yaroslav F., Dmitry Popov, Christian Riekel,b Gerard Ferey,† and Christian S.How Linker’s Modification Controls Swelling Properties of Highly flexible Iron (III)-Dicarboxylates MIL-88 J.Am.Chem.Society. 2011
Ravikovitch, P. I.; Neimark, A. V. Density Functional Theory Model of Adsorption Deformation. Langmuir 2006, 22, 10864 −10868.
Remy, T.; Baron, G. V.; Denayer, J. F. M. Modelling the Effect of Structural Changes during Dynamic Separation Processes on MOFs Langmuir 2011, 27, 13064 −13071.
Sarah Couck Department of Chemical Engineering (CHIS), CO2 capture and separation using metal-organic frameworks: effect of amine functionalization and framework flexibility separation of CO2 from gas mixtures.2012
Savonnet, M.; Bazer-Bachi, D.; Bats, N.; Perez-Pellitero, J.; Jeanneau, E.; Lecocq, V.; Pinel, C.; Farrusseng, D. Generic post functionalization route from amino-derived metal-organic frameworks. J. Am. Chem. Soc. 2010, 132, 4518–4519.
Serre, C.; Mellot-Draznieks, C.; Surble, S.; Audebrand, N.; Filinchuk, Y.; Ferey, G. Science 2007, 315, 1828.
Sugiyama, H.; Watanabe, S.; Tanaka, H.; Miyahara, M. T. Adsorption-Induced Structural Transition of an Interpenetrated Porous Coordination Polymer: Detailed Exploration of Free Energy Profiles. Langmuir 2012, 28, 5093.
Tan, J. C.; Cheetham, A. K. Mechanical Properties of Hybrid Inorganic −Organic Framework Materials: Establishing Fundamental Structure-property Relationships. Chem. Soc. Rev. 2011, 40, 1059 − 1080.
Tan, J. C.; Civalleri, B.; Lin, C.-C.; Valenzano, L.; Galvelis, R.; Chen, P.-F.; Bennett, T. D.; Mellot-Draznieks, C.; Zicovich-Wilson, C. M.; Cheetham, A. K. Exceptionally Low Shear Modulus in a Prototypical Imidazole-Based Metal-Organic Framework. Phys. Rev. Lett. 2012, 108, 095502.
Trung, T.K.; Trens, P.; Tanchoux, N.; Bourrelly, S.; Llewellyn, P.L.; Lorea-Serna, S.; Serre, C.; Loiseau, T.; Fajula, F.; Férey, G. Hydrocarbon Adsorption in the flexible metal-organic frameworks MIL-53(Al, Cr). J. Am. Chem. Soc. 2008, 130, 16926–16932.
Wang, Z.; Cohen, S.M. Post synthetic modification of metal-organic frameworks. Chem. Soc.Rev. 2009, 38, 1315–1329
Xiang Z., Z. Hu, D. Cao, W. Yang, J. Lu, B. Han, W. Wang, Angew. Chem., Int. Ed. 50 (2011) 491.
Yazaydin A.O, A.I. Benin, S.A. Faheem, P. Jakubczak, J.J. Low, R.R. Willis, R.Q.Snurr, Chem. Mater. 21 (2009) 1425.
Yoon, J. H.; Choi, S. B.; Oh, Y. J.; Seo, M. J.; Jhon, Y. H.; Lee,T.-B.; Kim, D.; Choi, S. H.; Kim, J. Catal. Today 2007, 120, 324.
Zou R.Q, A.I. Abdel-Fattah, H.W. Xu, Y.S. Zhao, D.D. Hickmott, CrystEngComm 12 (2010) 1337.