Abstract
Sustainable building materials and technologies, which are used in the construction and building industry provide solutions for addressing the problems of discharging waste products and materials into the environment. The process of converting raw materials into finished products, including the conversion of raw materials to cement and concrete consumes large amounts of water, air, and energy, which ultimately results in toxic discharges into the soil, water, and air systems. Such a scenario provides the rationale to explore a wide range of sustainable materials for use in the building and construction industry. A study of the literature on the characteristics of the materials for sustainable site development and the technologies to use provide the basis of this study. The study focuses on the use of the qualitative research design to address the main research question based on an analysis of secondary sources, which provide qualitative information to answer the research question. The findings show a strong need to shift to the use of sustainable construction materials and to the reuse of waste products and to design modular structures to reduce the embodied energy responsible for polluting the environment. Suggestions show a lot of optimism in the use of new technologies for future sustainable construction.
Introduction
This paper focuses on the study of sustainable building materials and technologies, which are used in the construction and building industry. The aim is to discover the best materials and methods to use in the construction industry to address the environmental problems caused by pollutants and toxic wastes, which are produced during the processing and consumption of building products in the building and construction industry (Halliday 2008). Researchers including Halliday (2008, p.45) agree that most of the environmental problems are caused by processing virgin materials from the earth and the disposal of waste products in the construction industry. Huovila and Koskela (1998, p.12) argue that such processes have a significant impact on the environment, which includes the release of toxic substances into the air, water reserves, and soil. The problems are compounded by the disposal of wastes products and embodied energy, which is the direct result of processing raw materials into finished products for construction purposes. To address the problems, this research will use a qualitative research design, which is based on the grounded theory to investigate and provide solutions on the best alternative materials, which are appropriate for sustainable development. According to Jonkers, Thijssen, Muyzer, Copuroglu and Schlangen (2010, p. 45), the properties of different materials have been explored including materials for sustainable design, materials that can be reused, concrete and its products and the materials, which can be used to make sustainable concrete instead of the use of the current processes of manufacturing concrete to reduce embodied energy and the adverse effects on the environment. In addition, the paper will focus on different areas of study in the context of the inputs and outputs required to process construction materials and the impact the material has on human health and the environment. To address the study, a literature review of secondary sources will provide the information required for use to provide the answers to the research.
Objective
The main objective is to discover the best alternative materials, their properties, and the technologies for use in the construction and building industry to achieve sustainability for future construction projects.
Research Question
What building materials, their properties, and technologies are available for use in the construction and building industry, which could help the industry to achieve sustainability for future use in the construction and building industry?
Literature Review
Sustainable building materials
A research study by Ayers (2002, P.12) into the demand for building materials shows that the need for superior performance construction materials has facilitated increased research, development, and innovation into sustainable building materials. The study focuses on sustainable construction materials that are used in the construction industry in the context of financial viability, thermal efficiency, occupational health, environmental impact, and properties that make the materials renewable. According to Anink, Boonstra and Mak (2004, p.23), energy efficiency and low maintenance costs have raised the compliance requirements for high-quality construction materials. It forms the basis of the research into sustainable building materials. The study focuses on the properties of different building and construction materials, an assessment of their impact on the environment and other issues of sustainable building materials.
Environmental impact assessment
A study of the issues associated with sustainable building materials by Anink, Boonstra and Mak (2004, p.34) including the processing, manufacturing, and use of the materials shows a wide range of negative impacts the processes cause on the environment. The impacts include increased carbon emissions, disturbance and destruction of natural habitats, and the loss of topsoil when preparations are made to make structures. Contributions by Berge (2009, p. 10), on the effects on the quality of air, including increases in dust emissions during the construction process are some of the critical issues associated with sustainable building materials. This section will focus on the assessment of the environmental impact caused by the embodied energy, which is the energy consumed in the mining and production processes of construction materials (McDonough & Braungart 2002, p.34). The study begins by exploring the inputs and outputs of the impact of construction materials on the environment, which account for the key transformation processes of changing inputs in the form of raw materials into the outputs in the form of finished products for use in the construction industry.
Inputs and outputs
Typically, the input processes begin with the extraction of raw materials from the earth. The earth is the source of the raw materials and is the sink of the used products (Kibert, Sendzimir & Guy, 2001). The extraction process causes adverse effects on the environment by damaging the ecosystems, natural habitats, and other environmentally destructive effects. Those effects are caused by releasing toxic gaseous emissions into the environment. In addition, the movements of the raw materials have profound impacts on the environment because of the embodied energy, which is consumed when moving the materials from one extraction site to the processing site. Typically stone and other primary aggregates form the primary sources of materials, which are used in the preparation of concrete, which is the primary material used in the construction industry. It has been established that the transportation of raw materials causes a lot of noise pollution besides other effects such as dust emission into the environment. The same issues arise when disposing of the waste and used products to the earth as the sink of the byproducts (Kibert, Sendzimir & Guy, 2001).
Other inputs which have been established to have severe consequences on the environment include the consumption of large amounts of water and energy for the transformation of the raw products into the finished products (Thompson & Sorvig 2007). Here, when such consumptions occur, the net effects cause adverse impacts on the environment in the form of the discharge of solid wastes, toxic gases, and water, which are released into the environment and into the air. Here, it has been established that during the transformation processes, significant quantities of water, acid, and pollutant are released into the environment. In addition, the extraction process of raw materials discharges some substances with biological toxins, mutagens, carcinogens. Each phase of the extraction of the raw materials processing lifecycle causes a significant impact on the environment (Thompson & Sorvig 2007). The additional burden on the environment could raise extra costs, which underpins the need for policies to prevent the impact of such substances on the environment.
Human Health and Environmental Impact
The argument by Mehta and Burrows (2001, p. 3-23) shows that the lifecycle processing of raw materials and finished products provides additional challenges because of the adverse impact such processes cause on the environment and on human health. Here, there are intense interactions between the environment and the material production processes. The interactions between the environment and the earth occur in two ways. Here, there are two ways include a situation where the earth acts as the source of the raw materials and the second way is where the earth acts as a destination sink of the emissions, solid wastes, and affluence, which result from the extraction and use of raw materials and finished products (Mehta & Burrows 2001).
It has been established that construction materials form one of the greatest segments of the earth, which acts as the source and the sink of the materials and is the source of the effects on the environment. The manufacturing processes of the raw materials degrade the environment and the ecosystems at the source location of the raw materials (Mehta & Burrows 2001). The entire process leads to the increase of global temperatures, which has led to the rise in sea levels, reduced food supplies, displacement of populations, violent storms, and significant loss of biodiversity. Typically, greenhouse gas emissions lead to the average increase in global temperatures on the earth (Mehta & Burrows 2001). Here, greenhouse gas emissions have caused an increase in greenhouse gas concentrations in the atmosphere. The connection between the environment and construction materials has shown that global climate change is caused by emissions from energy use, which is the direct result of the burning of fossil fuels and non-fossil fuels, landfill processes, cement, iron and steel processing, and transportation of the raw and processed materials from one side to the other (Mehta & Burrows 2001).
In addition, there is a strong connection between fossil fuel depletion and construction materials, which is based on the usage of fossil fuels and electricity. Typically, the transformation process of the raw construction materials into the finished products consumes significant amounts of energy and consequently leads to global temperature increases. The loss of biodiversity is caused by the large consumptions of water, acid deposition, mining, dredging, thermal pollution, and resource extraction. Other environmental concerns associated with sustainable materials include smog. Smog is the direct cause of the combustion of fossil fuels, material mining and processing, demolition and transportation of the material and the waste products. Estimates indicate that the United States produced 94% of the CO2 emissions into the atmosphere and the trend of releasing greenhouse gas into the environment has been on the increase (Williams & Dair 2007). Research shows that 75% of the anthropogenic greenhouse emissions are the result of fossil fuels consumption from power plants and vehicles, which are the primary means of converting and transporting the raw materials, finished products, and waste products into the environment (Williams & Dair 2007). That necessitates the need for materials that have a minimum negative impact on the environment. Typical examples include certified wood, adobe blocks, glass, glazed bricks and ceramic bricks. In a study on the environmental impact of construction materials, it is critical to assess the impact caused by the materials for sustainable construction. One of the approaches is to focus on the assessment of the impact of the input and output on the environmental and human health impact on the people (Williams & Dair 2007).
McDonough and Braungart (2002, p.34) provide a comprehensive review of the input and output resources used to make construction materials. According to McDonough and Braungart (2002, p.34), the engineering procedures of processing construction materials lead to a complex impact on the environment. The adverse effects start from the process of mining the raw materials from the earth and returning the waste materials into the sink, which ultimately causes emissions, affluence, and solid wastes into the environment. The process causes material depletion in terms of quality, quantity, and ecosystems leading to an adverse impact and imbalance of the natural processes of the ecosystem due to the overuse of sources and sinks. One of the effects is climate change (McGowan & Kelsey 2003, p. 23).
The connection between environmental degradation and changes in the global climate is in the context of greenhouse gas emissions, which occur because of iron and steel processing, cement production, landfill processes, and the transportation of the products (McGowan & Kelsey 2003, p. 23). The processes cause the loss of biodiversity during the extraction, manufacturing and discharge of the wastes. In addition, acidification due to the emission of sulfur and other exhaust gases, and emissions due to the drainage of mines cause further environmental degradation. Other sources of adverse environmental effects include acid leaching, exhausts from fossil fuel combustion, ecological biodiversity, smog, and stratospheric ozone depletion (Berge 2009, p.5).
The critical issues of sustainable building are in the acquisition, processing, and transforming the inputs of construction materials into outputs. Here, inputs are in the context of the acquisition phase, which demands the use of energy to drill, mine, dredge, and harvest the inputs. In that context, the environmental impact includes the destruction of natural habitats at the points of extraction of raw materials. If the primary materials or the ore wastes are toxic such as materials that oxidize upon exposure to the air resulting in acid mine drainage, or soil erosion that causes sedimentation and blocking of waterways, the result can be disastrous.
The process of refining and refining and processing of primary materials is another element of sustainable materials (Mendler, William & Mary 2006, p.64).
Water resource depletion
Water resource pollution and depletion are some of the serious effects that have been demonstrated to cause very serious effects on the environment. The rationale for the depletion and degradation of the water as a resource is because the transformation process of converting raw construction materials into finished products such as concrete and cement consumed significant amounts of water (Williams & Dair 2007).
Concrete
Concrete is a material that is widely used in the construction industry in the world, which consumes over 1m million tons of cement annually. Concrete is popularly used because of its strength when appropriately mixed in the right proportions with water, admixtures, aggregates, cementitious and pozzolanic materials (Mendler, William & Mary 2006, p.64). In addition, 1 billion tons of water, and 10 billion tons of rock and sand are consumed annually. The cement production process requires that for each ton of cement, 1.5 tons of fossil fuels and limestone are consumed. It has been established that the high popularity of concrete in the construction industry has accelerated its use and the environmental costs associated with its use in the construction industry. Here, the consumption of energy to produce concrete is one of the sources of the adverse impact on the environment (Williams & Dair 2007). Studies show that the concrete aggregates cause significant environmental impacts, which include water and other resources. In addition, other areas of concern include the adverse effects on the atmosphere and the environment, which are classified into the background noise from machinery and plants, intermittent noise from blasting and screening, crushing, and moving vehicles. In addition, the water environment is another area of concern (Williams & Dair 2007). Typical disturbances include queries, which lead to the modification of the flow of groundwater and water quality. In addition, the modification of runoff quality and quantity of water and increase in the sedimentation contributes to the adverse effects on the environment (Williams & Dair 2007).
The huge consumption and water and energy to produce concrete comes with huge environmental costs such as CO2 emissions, energy consumption in the processing and transportation of the raw materials and other costs are some of the issues associated with the production of concrete(Williams & Dair 2007). That leads to the study of the environmental impact of concrete components discussed below. Research studies have shown that the four-step process of producing cement and concrete significant amounts of energy and water and leads to the release of large amounts of wastes into the environment (Williams & Dair 2007). The lifecycle process of working on the production of cement leads to dust emissions, which are discharged in great quantities into the environment. Here, there is a significant tonnage of materials involved in the production process, with a substantial impact o the environment (Williams & Dair 2007). Here, the manufacture of clinker, which is one of the critical components of manufacturing Portland cement, produces large amounts of CO2. Here, there are other particulate emissions, which make a significant contribution to the environment, which result from quarrying activities on the point of source, production, and discharge of the waste products into the environment. It has been estimated that fugitive emissions which are of course particulate in nature are not only a nuisance but also a health risk (Williams & Dair 2007). The particulate nature of the products is classified as PM10 and PM2.5, and is in the range of < 2.5 µm and. 10 µm.
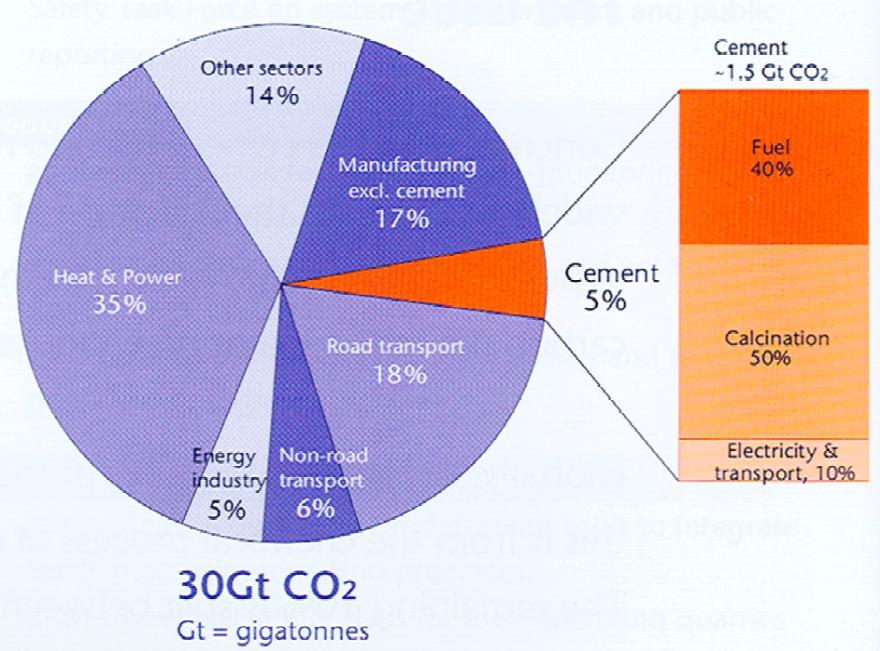
The graph above shows that the amount of carbon dioxide contributions to the environment due to the production of cement is 17% of the total global carbon emissions. In addition, the environmental profile of steel-reinforced concrete is shown in the map below.
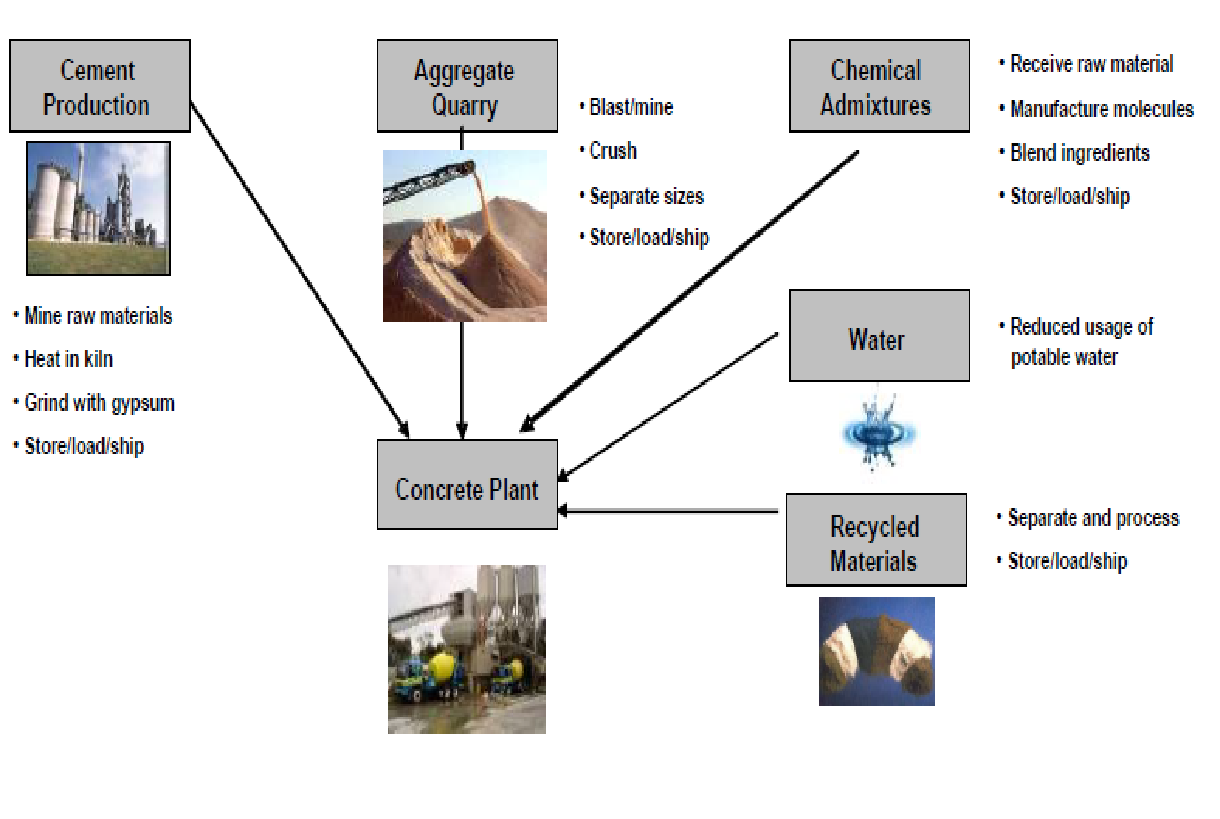
Other environmental burdens on the use of concrete include the process of land use and the process of procuring and processing the raw materials, which are used in the production of cement, and the energy used in those processes. Some of the examples are shown below and are rarely related to the emission of different exhaust materials and energy used in the production of slabs.
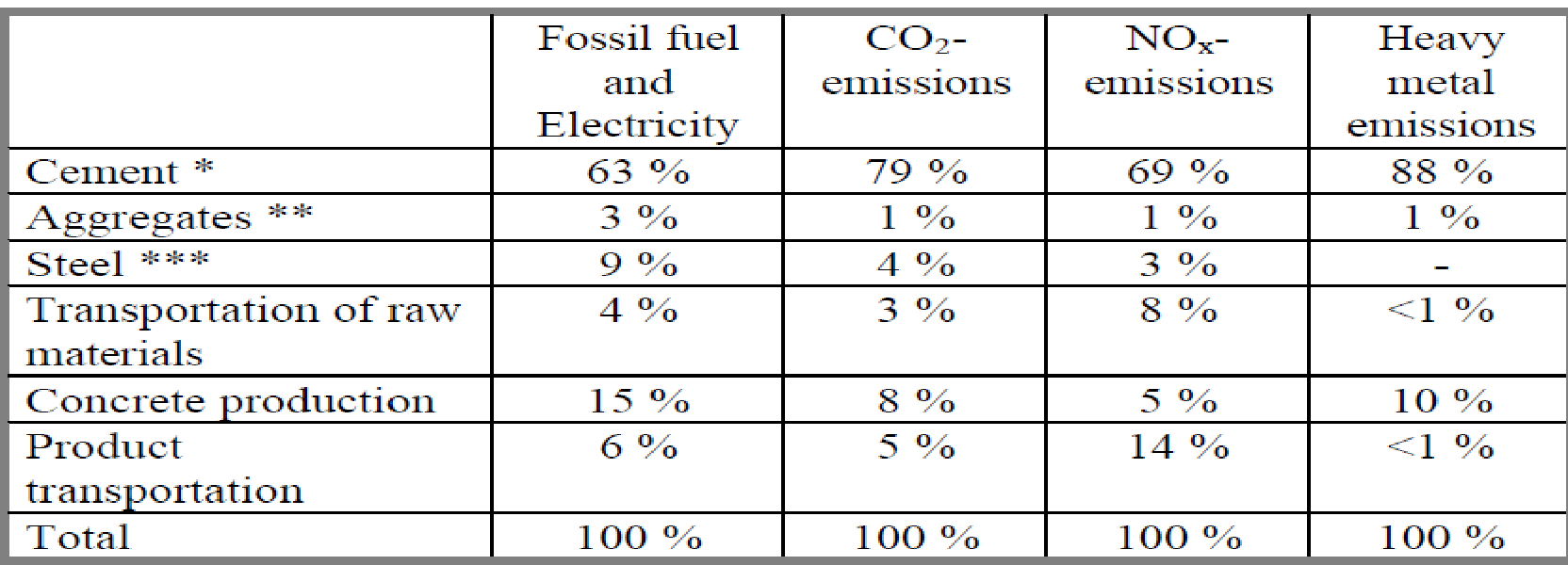
It is easy to see that the production of the concrete slab consumes cement, which accounts for 63% of the energy consumption in the form of fossil fuels and electricity, 79% carbon dioxide related gaseous emissions, 65% nitrogen oxide related emissions, and 88% emissions of heavy metals into the environment. On the other hand, there is the consumption of aggregates, which constitute 3% of the fossil fuels and electricity consumption and 1% carbon-related emissions. Other areas, which contribute to the consumption of energy, which has ultimate impacts on the environment include product transportation, concrete production, and the transportation of raw materials. The entire process of producing cement consists of the procurement of raw materials, the production of cement, and the movement of raw materials into the processing site.
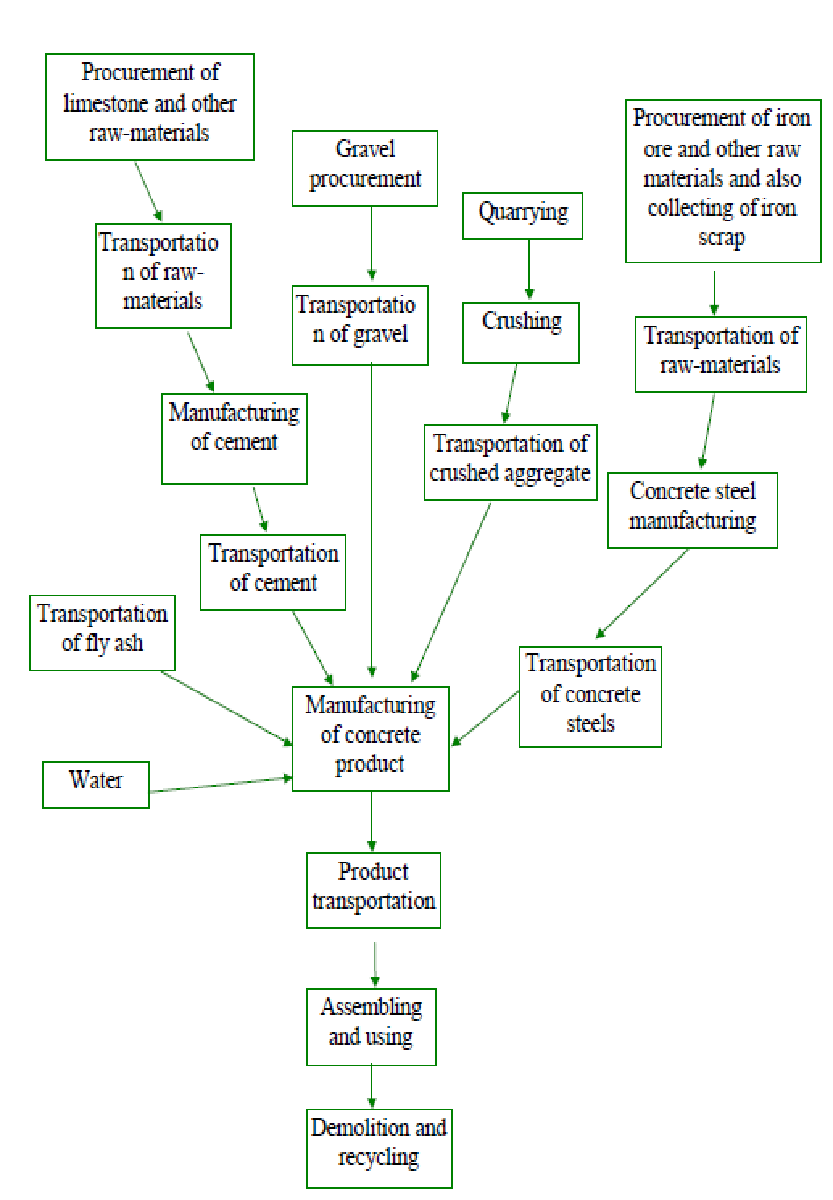
Portland cement
According to Calkins (2012 p.76), cement is the main component used to manufacture concrete, with the production process causing the most adverse impact on the environment. The concrete industry consumes significant amounts of energy with the global intensity being the highest per dollar value of the outputs in the manufacturing of cement. According to Calkins (2012 p.29), the critical components for making cement in a four-step process that consumes significant amounts of energy include clay, shale, limestone, and rock or marl in varying concentrations. During the four phases of, cement manufacturing process large amounts of energy are consumed releasing large amounts of CO2, kiln dusts with an industry estimate of 38.6 kg for each metric ton of cement, other wastes from the burning of coal in the energy-intensive manufacturing process. Air emissions include particulate matter, carbon monoxide, sulfur oxide, nitrogen oxides, hydrogen chloride, and other hydrocarbons, which are emitted during the different types of concrete producing processes, which have emission levels of 5% of the global carbon emissions (Calkins 2009, p. 18).
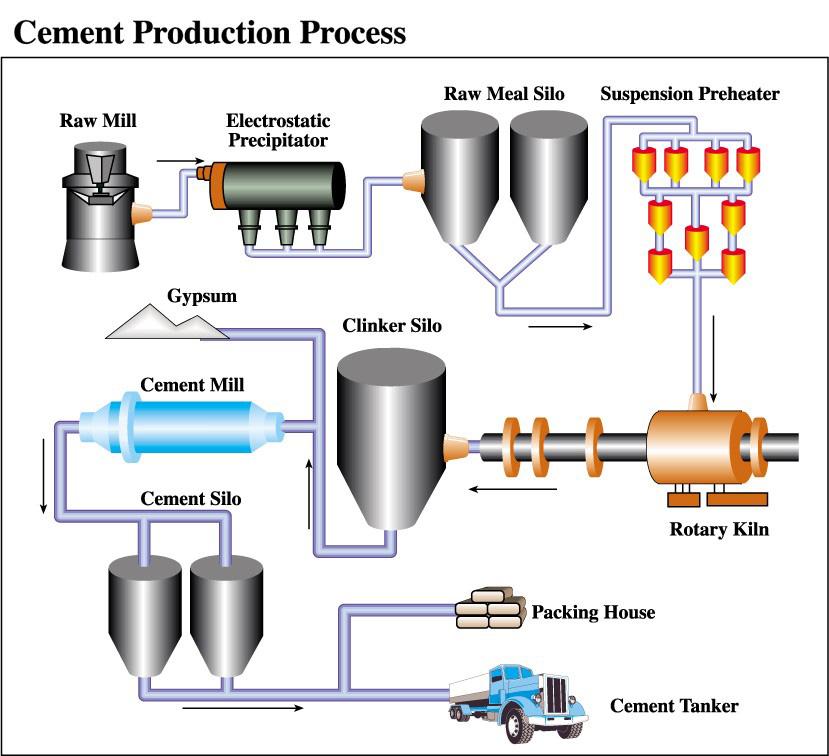
Energy use in the production of cement occupies a significant space in the production of materials that cause adverse effects on the environment. Typically, the effects are in the quarrying and crushing processes of the raw materials, the storage of raw materials, the processing of the raw material when grinding and blending them, the production of clinker, which is a critical component in the production of cement, finish grinding of cement, and when packaging the cement (Peris 2007). The most environmentally devastating phase is in the preprocessing stage where clinker and kiln exhaust stacks emit toxic substances into the environment. Here, the production of other substances, which are classified as particulate matter or PM particles constitute some of the disastrous effects on the environment, which need some radical thinking and shift toward the use of sustainable building materials (Horvath 2004). A critical analysis of the other sources of PM materials shows that they occur in low storage piles and during the loading and unloading processes within the facilities, which are facilitated by the transformation processes of converting them.
The environmental burden of the production of cement is shown in the diagram below.
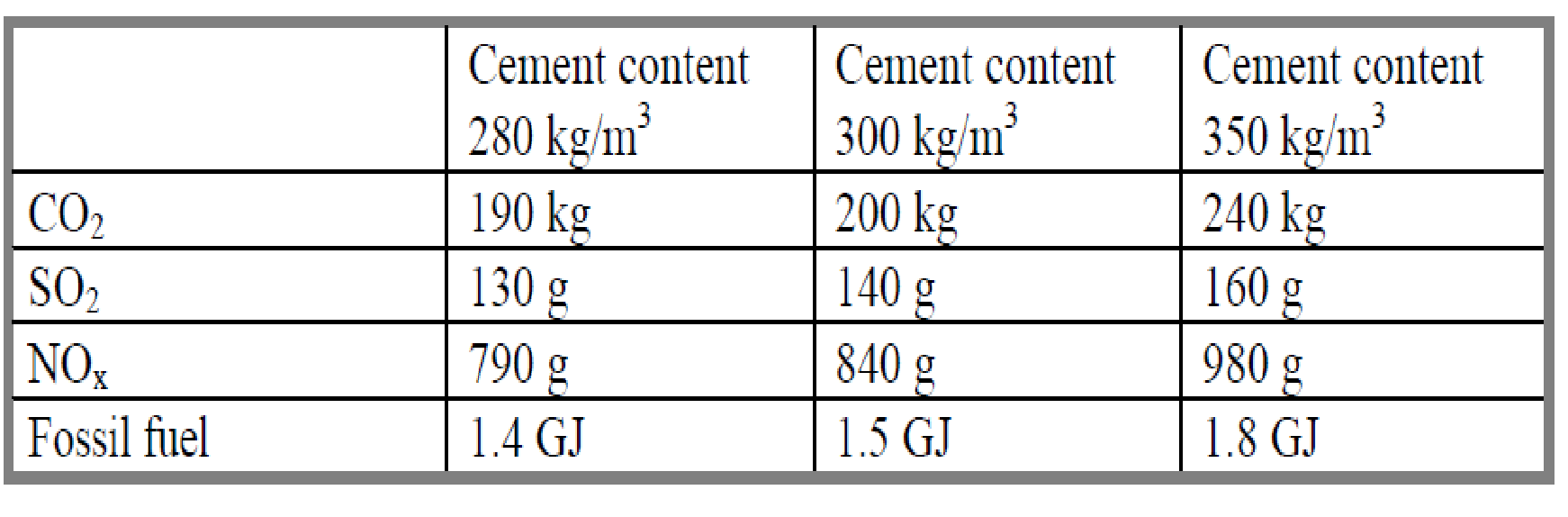
In summary, the production of cement and its relationship with the environmental burden is based on the fact that cement constitutes up to 15% of the concrete and is the greatest pollutant among the aggregates used to make concrete. The environmental burden is caused during the extraction of raw materials used to make cement, the transportation of the materials, and processing, which consumes significant amounts of energy (Horvath 2004). During the transformation processes, large amounts of energy are consumed to decompose certain materials such as calcium carbonate, which makes up to 60% of the emissions released into the environment.
Minimizing adverse impacts
Current programs to minimize the current and future impact caused by the production and consumption of cement and concrete include efficient use of innovative cement products, use of high-performance cement, and building stable structures to efficiently utilize concrete and its mixtures (Calkins 2012, p. 28). Several suggestions have been made, accompanied by the evidence on the alternative approaches and use of other materials to reduce the impact of concrete and cement on the environment. One of the evidence-based suggestions is to use cement substitutes. Cement substitutes, which consist of industrial by-products include GGBFS, which is slag that can cool very fast when exposed to the environment. The substance has been proved to work very well to improve the workability, durability, and strength of concrete. The advantage of this material is that it provides chloride permeability characteristics to the concrete and makes it has good heat hydration capabilities. It has been demonstrated that the material makes concrete possess additional comprehensive and flexural strengths and is able to mitigate the effects of sulfate attacks, which come from the dry land or from the seawater (Horvath 2004). It is possible to remove the effects of pozzolanic activities using GGBFS, which is found in high silica aggregates. The net results are that the resulting concrete is lighter and provides better sources of concrete, which is environmentally friendly. The only drawback in the use of GGBFS is that it is slightly expensive because of the additional costs of shipping the substance (Horvath 2004).
Fly ash is an industrial by-product, which can be used to substitute natural products, which are used in the production of concrete (Horvath 2004). It has been demonstrated to be critical in preserving the environment from the destructive effects of natural substitutes (Horvath 2004).
One process that has proven energy efficient is the use of blended concrete. Blended cement, which is made from fly ash, silica fumes, clinker, and natural pozzolans allows for a reduction in embodied energy. The advantages of blended cement include higher production capabilities, lower waste emissions, and reduced energy consumption. A shift in the use of dry processing methods introduces the problem of energy consumption in the use of preheaters (Calkins 2012, p. 18).
Concrete can be made from any of the two types of fly ash. One type is the class c fly ash. Fly ash is produced when sub-bituminous coal with a 30% CaO content and silica iron, and alumina are burned to produce cementitious pozzolanic which requires hydrate and water to be produced. The resulting substance is energy efficient and offers excellent performance in terms of workability, long-term strength, and short-term strength strain. Another category of fly ash is Class F fly ash. Class F fly ash contains a low amount of calcium which is sufficient enough for the hardening process.
Fly ash makes significant contributions to the sustainable production of building materials because of the range of benefits gained from the use of the material. Advantages include improved workability by reducing the amount of water consumption, high performance and workability because of the electrostatic effect of the cement particles, and better pumping and consolidation capabilities. Increased set time allows for more time to work on the concrete which increases the quality of the concrete. Increased set time leads to the higher compressive strength of the concrete. One shortfall with this approach is the increase of the time required for concrete to set and gain its appropriate strength, above the 28 days required for the process to enter into completion. However, the procedure results in higher cement savings, despite the 56 days required for the concrete to be set.
Research has shown a number of materials that can be used as a substitute material for the production of concrete admixtures. One of the methods is to use recycled materials for virgin aggregates. The aggregates have environmental, aesthetic, and economic advantages. The critical advantages translate to reduced embodied energy by reducing the mining and processing of the raw materials, reduction in environmental impact due to mining of the raw materials, and the reduced effect of landfill problems. The table below illustrates the substitutes with the above-mentioned advantages in the production of concrete, the performance benefits, drawbacks, and the percentage of substitutes for each substitute.
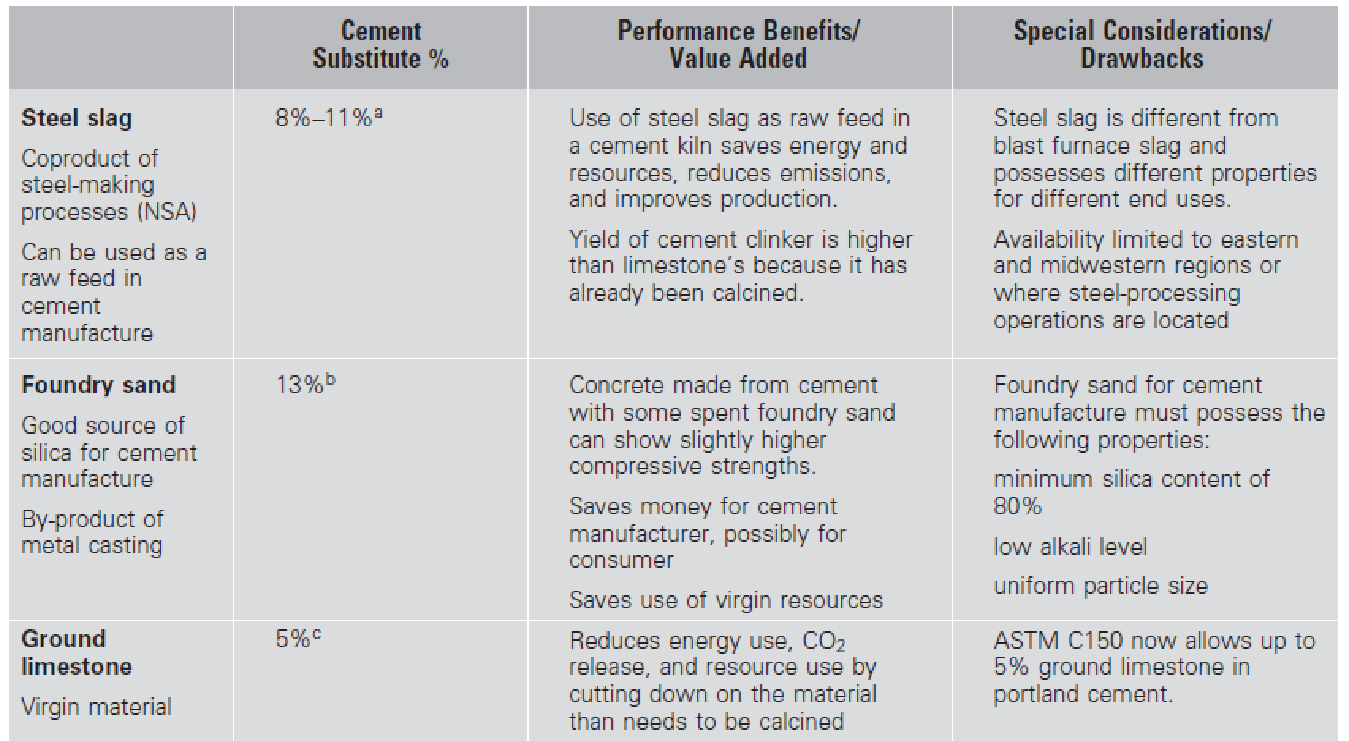
It is critical to note that there are a variety of other recycled products that can be used as substitutes in the economic and environmentally friendly production of cement and concrete. However, the primary limitations of using recycled materials are the limitation to predict the performance, the ability to predict the performance of the resulting concrete. It is also critical to note that it could also be difficult to predict with precision the water absorption, compressive strength, and specific gravity compliance to standard requirements and accurately predict the properties of resulting concrete (Norton 1997, p. 90).
The critical benefits associated with the use of recycled materials, that address issues of sustainability and impact long term adverse impact on the environment include lost costs of production, reduced energy consumption and higher energy efficiencies because of the reduced need to transport the aggregates because most of the recycled materials are used on site. However, the issues and challenges associated with recycled materials include production of dirt, fluctuating quality and performance of recycled materials, and the challenges associated with making the appropriate concrete mixtures.
Another material that is worth discussing is the use of reclaimed concrete aggregate (RCA). RCA can be used in new structures as fine and coarse materials. The use of RCA has been shown to be money-saving and is one approach that can be sue dot save the virgin environment. It has been shown to be suitable for use in urban areas and where gravel is not easily available. One strategic approach used to acquire RCA is through the recycling of concrete structures on site. It is possible to bring the stone crushing equipment on-site to ensure that little energy is spent in the transportation of the concrete wastes to the processing site (Horvath 2004). The typical process of recycling concrete is to crush it on the site after the removal of the old concrete from the target site, then crushing the old concrete and removing steel and other embedded items (Horvath 2004). After the process, the resulting concrete is graded, washed, and stockpiled into fine aggregates. Research evidence shows that the use of reclaimed concrete presents additional challenges because it is possible to be contaminated with a significant number of dirt, foreign materials, debris, and other additional materials. The RCA has to be graded and additional water added to ensure that the RCA gains the desired comprehensive strength of concrete for construction purposes.
Crushed recycled glass is another substitute material to use as a fine aggregate to make concrete. The main challenge of using glass as an aggregate material is the presence of alkaline substances, which when they react with concrete create additional substances which swell and crack the concrete. However, the adverse effects can be overcome by using glass which has been ground to particulate o very fine powder and the use of additional aggregates, which reduce the activity rate of glass with concrete (Horvath 2004).
It is possible to use concrete admixtures to impart special properties to concrete during the preparation of concrete. The use of admixtures alters the properties of concrete in terms of workability, curing temperature, color, permeability, and set time. Little documentation has been provided of the human health effects in the use of admixtures, but a number of admixtures have been developed. Those admixtures include the air entertaining admixtures, which improve the properties of concrete when in freeze-thaw conditions, and the workability of the concrete. Water reducing admixtures necessitates the use of less water when preparing the concrete and have been shown to be suitable for use in hot weather placements.
Other strategic approaches to ensuring that materials for sustainability have been developed and used include the use of decorative concrete finishers (Hammond & Jones 2008). Here, the agents used are of various colors and can be used to save a lot of resources, which could be used to finish the concrete in terms of energy and money (Hammond & Jones 2008).
The use of concrete sealers has been recommended to be another strategic approach to reducing the environmental impact caused by the use of concrete. It is important to use the sealers sparingly to reduce the adverse impact such sealers have on the environment because they contain hazardous materials, which have adverse effects on human health (Hammond & Jones 2008). In addition, it has been established that concrete sealers can contain very hazardous chemicals, which can have an adverse impact on the quality of air, water, and soil (Hammond & Jones 2008). Here, it necessitates the use of sealers, which are made from organic biodegradable materials as sealants to avoid the use of toxic materials which have an adverse impact on the environment. It is important to ensure that concrete structures are adequately reinforced to ensure that they last for a long time to avoid regular maintenance and construction of new structures. The ultimate aim is to limit or reduce the amount of embodied energy used in the construction of new buildings, which translates into the energy absorbed when new materials are extracted and processed to create the new products..
Another material that has been suggested for use to address the issues of sustainability is porous concrete. Porous concrete can be used instead of using concrete paving to reduce stormwater management in cities. Research shows that stormwater carries with it a high concentration of chemical wastes and other pollutants (Hammond & Jones 2008). Porous concrete is highly permeable and allows water to permeate into the ground slowly. The net result is that stormwater is filtered while penetrating into the ground. The process allows clean water to drip into underground water, which is otherwise contaminated by the stormwater, which flows into the underground water reserves. Porous concrete contains aggregates, which are uniformly arranged to enable the seepage of water without the particles, which make the water dirty and full of contamination from toxic substances (Hammond & Jones 2008).
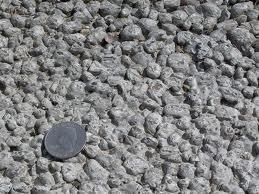
Design for sustainability
Engineers and architects have embarked on developing energy-efficient concrete and cement processing technologies. In addition, the designers and planners should embark on the development of structures based on the principle of “design for sustainability” which is based on the principle of mutual respect or the people and the environment (IEA. 2010b, p.45). The principle stipulates that developers use locally available or indigenous materials for construction purposes, use efficient construction methods to preserve open spaces in cities, and endeavor to create designs and embark on construction processes that protect and create rich topsoils (IEA 2010b, p.45).
It is critical to consider the entire lifecycle of any structure to ensure effective environmental preservation is put into practice (Minke 2006, p.23). Typical construction processes should include effective and efficient extraction methods, processing, transportation, and construction technologies and materials that are sustainable when being processed. In addition, any solid wastes that cannot be processed further should be eliminated or dealt with in accordance with toxic waste disposal methods. It is important to study the lifecycle of the material to be used before adopting the use of the material. The critical points to study include the costs associated with the mining, processing, and transporting of the material to the construction site (Minke 2006, p.23).
Other elements to include in the study is the best and most energy-efficient method of mining, processing, transporting, blending, and converting waste materials back into the environment. It is important to make designs that use less water for construction and do not lead to environmental degradation. Typically, the design process includes the ability to identify those materials, which have a long service life and can be reused once the structure they have been used to set up is demolished or dismantled. In addition, it is important to ensure that the products measure up to standards required for construction products so that they can easily fit into other structures without being reworked. In addition, the importance of using modular design specification is a critical step in ensuring that once a structure reaches the end of its lifespan, most of its components can be reused. It is also possible to make flexible designs, which can be altered without making much and costly changes to address emerging needs (Hammond & Jones 2008).
Design for environment
Designing for the environment is one of the strategic approaches used in the design of structures, which cause minimum impact on the environment. The thinking here is to consider and factor issues related to industrial ecology and environmental preservation into the design of products, and especially structures in the construction industry (Huovila & Koskela 1998). A typical balance of technical considerations and business factors provides the designer with the bests design for achieving ecological balance. The design strategy is to design for disassembly and assembly of the construction product. In addition, the design for assembly and disassembly is based on the concept of dematerialization, which is based on the concept of less wastage and more goods.
The goal of the design for the environment (DFE) is to ensure that every input element into the production process should exit a system as a product, which can be sold. The concept further requires that every transformation process should only use the required amount of energy (Huovila & Koskela 1998). It is recommended that industries should use the minimum amount of energy in transforming processes of inputs into outputs, products and services to avoid the waste of energy. The core principle of the design for the environment requires that the use of recycled materials be taken as the best option instead of using raw materials, which require the use of large amounts of energy to transform into the finished products. It has also been suggested that products should be created to be useful at the end of their lifecycle. In addition, the embedded utility of the products should be retained during the construction process.
Another approach is to use the concept of closed-loop systems and zero waste discharges. The closed-loop system is where a product is used continuously to ensure that even if it reaches the end of its lifespan, it has to be reused again and again in different forms. The rationale is to ensure that the material is used and reused to avoid creating wastes and polluting the environment. One of the philosophical approaches to the use of the concept is the cradle-to-cradle design where materials used for construction are designed with the aim in mind of making them reusable to avoid wastes, which pollute the environment. The model proposes the creation of materials, which produce wastes, which are beneficial in every phase of the product’s lifecycle (Huovila & Koskela 1998). Here, the closed-loop system provides zero wastes because every input or output is valuable and can be used at every step of the product lifecycle. Here, changes to product design involve creating a product for sustainable reuse to avoid product obsolescence. Here, the principles of green chemistry play a significant role in the production of sustainable materials.
Here, the chemical synthesis of products should prevent waste. The chemical synthesis process should not leave any wastes and or clean up. It is critical to design critical chemicals, which do not create toxic products into the environment and which are less hazardous to human health and to the environment. In addition, it is critical to use catalysts to reduce chemical wastes in the environment.
Another approach that has been advocated for sustainability purposes is the extended producer responsibility (EPR). The principle calls for the manufacture of the product to ensure that they ensure responsibility for the entire life of a product. The aim is to reduce the number of wastes discharged into the environment by requiring manufacturers to ensure that they take the responsibility of designing products, which adhere to the principles of closed-loop systems. In addition, certain products can be repacked in their old packages and taking back the packages is one of the sustainability principles that can be embraced to ensure less waste is discharged into the environment (Hammond & Jones 2008).
It is important for other countries to follow the example of the European Union, which has created a number of legislations, which emphasize the reduction of waste. The legislations extend the producer’s responsibility in ensuring that the products are recovered and reused at the end of the product lifecycle. In addition, the implementation of the polluter pay principle is another approach that can be reinforced by legislation to ensure that organizations or anyone responsible for producing toxic wastes take full responsibility for dealing with the wastes. Research shows that the polluter pay principle is implemented with landfill taxes. There is a requirement in certain states in the United States, which require demolitions and other landfill wastes to be disposed of in special places designated for the disposal of debris.
Another method of ensuring that less energy is used in the construction of new buildings is the use of precast units, which are joined together using bolts and nuts when a new building is being set up. Later, when the same structure is demolished, the precast units can be removed by carefully dismantling them to remove the precast units which can be put to use in constructing a new building. Some countries such as the Netherland provide excellent examples of constructive demolitions. In the Netherlands, buildings are dismantled carefully and the recovery levels of building materials from such buildings are extremely high. In the Netherlands, construction systems are developed such that structures, which have been constructed from concrete, are dismantled and delivered to another site for use with very little input of new materials Huovila & Koskela 1998). A typical example of a structure that can be dismantled and assembled at the destination point with little input from new materials is shown below.
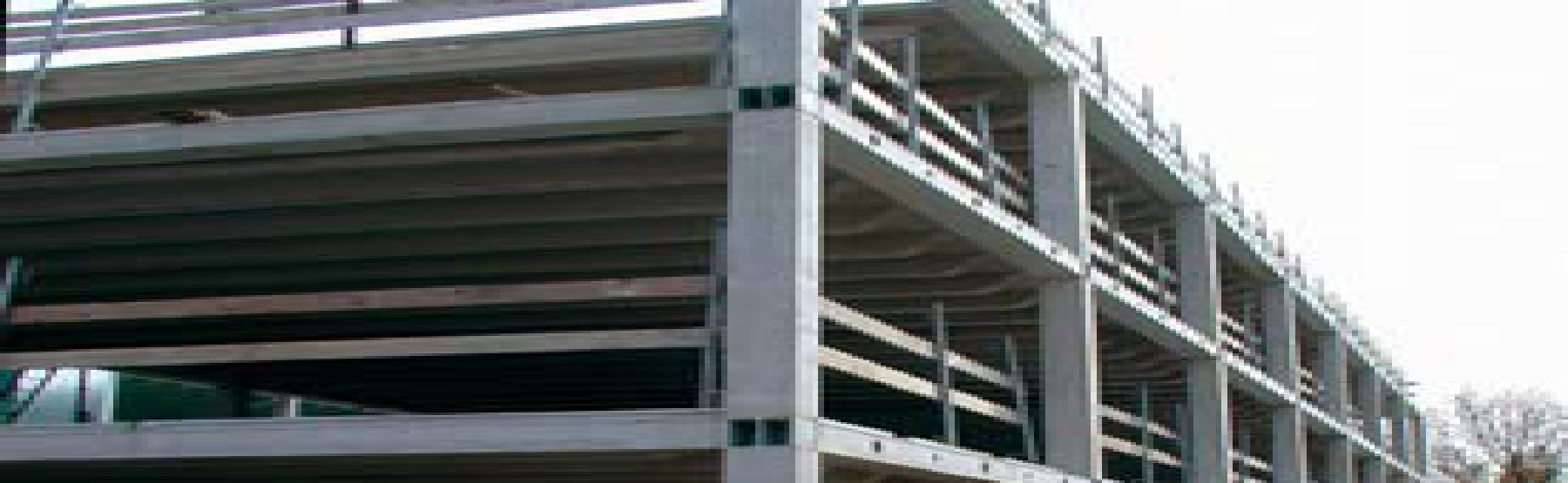
Sustainable building technologies
The use of sustainable building technologies provides improved solutions to the problems associated with adverse environmental impact, with the critical issues associated with the technologies including alternative building technologies, energy, and environmental issues. That is because building practices have dynamically evolved with time, leading to new the development of new technologies. Sustainable building technologies provide advantages which include sustainable energy consumption, reduced consumption of high energy materials, environmental energy-friendly technologies, and use of local skills, decentralized production of materials, optimal production and use of building materials, and the use of renewable energy sources (Norton 1997, p.12).
General conservation techniques provide the basis for sustainable building technologies. It is critical to note that the “layout and design and layout of a building are important in the conservation of energy” (Minke 2006, p.198). It is important to minimize the amount of excavation during the construction of a building to reduce the amount of energy consumed in the construction processes Huovila & Koskela 1998). Here, utility bills can be reduced significantly and the use of efficient technologies enables the users to address the issues.
One approach used in the production of energy-efficient materials and sustainable technologies is to optimize local skills while investing in future research and development. Some of the sustainable building technologies include the use of stabilized mud blocks, fine concrete blocks, steam cured blocks, soil lime plaster, Rammed earth blocks, and Lime–Pozzolana cement. According to the research, composite mortars for masonry and improved roofing systems which use Ferrocement and ferroconcrete are some of the technologies that are in use today (Norton 1997, p.19). The critical features of the technologies and issues associated with the technologies are discussed below.
Alternative Construction Materials
Stabilized mud blocks have been in use for the construction of low-cost buildings or structures with forces applied on the materials being significantly low. In this case, “machines compacted soil create dense solid blocks using sand and soils” (Norton 1997, p. 90) and the process is done using stabilizer to achieve the required mechanical properties” (Norton 1997, p. 90). The soil composition is the key determining factor of the compressive strength of the resulting block. It is possible to improve the quality and compressive strength of the block using additional stabilizers. The production process of the stabilized mud block is low embodied energy when compared to other bricks.
Another material is the use of filler slab roofs. Filler slabs are made from the use of filler materials which are cheap and lighter compared with materials for similar construction work. However, a “number of alternative materials can substitute the filler materials used in the construction of filler slabs” (Minke 2006, p.29). The quantity of the filler material used for substitution purposes depends on the area of application and the thickness required for the specific areas of application. Typical examples include a situation where 25% of concrete can be replaced to accommodate the substitute material in the construction of filler block measuring 60-70 mm.
Another substitute material used in the construction industry for sustainability purposes includes un-reinforced masonry vaulted roofs. Using the material yields additional benefits which include low production costs, low embodied energy, and better aesthetic values. In addition to that, the Lime–pozzolana cement, which is made from secondary grade lime and calcium hydroxide in the correct proportions provides the best substitute material for construction purposes.
It is important to use materials produced from renewable sources of energy because such materials cause minimum environmental impact. It is critical to conduct a study on the embodied energy of both alternative sources of materials based on energy sources. A typical example is an aluminum which requires a significant amount of energy for production purposes. When compared with steel, aluminum consumes eight times more energy than cement. However, aluminum consumes electricity from hydroelectric power sources while cement consumes energy from the burning of coal and related materials.
The use of eco-efficient construction and building materials is a concept, which was recommended in 1991 and provides the rationale for the development of products and services, which lead to the reduction of the costs of producing building materials. The concept has become popular in the construction industry because of the rapid depletion of raw materials and the adverse impact such depletion has on the environment Huovila & Koskela 1998). The choice of materials that are used for sustainable construction include should bear the specific focus on nontoxicity, low energy embodiment, high material durability, materials obtained from renewable sources, and materials, which can accommodate other waste products from other industries.
Earthen materials
Earthen materials have been in use in different parts of the world for a long. They consist of a variety of materials, which can be modified to meet the structural strength required for the construction of building materials. Such materials provide a significant number of benefits to the environment. Typically, the raw earthen materials are inexpensive
A survey of existing raw material used to manufacture construction materials that exist in the earth’s crust have different mechanical properties than can be exploited for construction purposes. The earthen raw materials incorporate soil to a significant extent, water, and clay. However, the properties of soil vary depending on the application and performance requirements (Norton 1997, p. 90).
The most appropriate soil for construction purposes includes silt, gravel soil, silt, and sand. Clay is a sticky material when made wet with water and its particles measure 0.005 mm and have strengths, which vary between high and medium plasticity. Some soils have mechanical properties, which are unsuitable for construction purposes (Norton 1997, p. 90).
Other types of soils include sand with high strength and low particle porosity. In addition to that, silt is another type of soil with low plasticity and mechanical strength, making the silt unsuitable for construction purposes. Gravel, which has low particle porosity, is appropriate for earth construction, especially applied in trench construction. It is important to improve the mechanical properties of soil to make it appropriate for earth construction purposes. One of the strategies used to improve the mechanical properties of soil to make it suitable for different construction purposes is densification. Densification is achieved through compaction, reducing the capillarity of soils pores, and eliminating any air in the soil (Norton 1997, p. 90).
The mechanical properties of soil can be achieved through segmentation using insoluble synthetic hair strands fly ash, and other materials already discussed in the paper. Other methods of improving the quality of soil include the use of linkages and imperviousness stabilizers. Impervious materials stabilize soil for construction by removing water and other ingredients. In addition, waterproofing is a technique that enables soil with the ability to resist the flow and penetration of water into the block (Norton 1997, p. 90).
The testing of earthen material should form part of the evaluation process of sustainable construction materials, which includes the need to determine the properties of soil and other earthen materials. The right composition of materials has to be determined to decide the correct composition of soil and moisture content in the construction material to ensure the mechanical; properties meet the required mechanical properties. Here, the mechanical properties vary depending on the area where the product is applied. One of the testing strategies includes quality control testing is done to ensure that the earthen materials perform according to the specified mechanical properties, which include modulus of rapture, comprehensive strength, moisture absorption, and resistance to water erosion.
Various methods have been used to stabilize soil for earth construction, which are achieved through a number of steps. Those steps include densification, which includes the processes of grinding and compaction to remove any traces of air, which have certain properties such as effective capillarity.
Energy consumption
Energy is a critical component in the manufacturing and production of cement. According to research studies by Minke (2006, p.29), energy for the “construction of buildings can either be in the form of embodied” ” (Minke 2006, p.30) and “energy or in the form of energy consumed to maintain a building” (Minke 2006, p.31). Energy consumed to maintain a building depends on changes in the climate and other variations in the weather. The following table illustrates energy embodiment for different rent walling and roofing systems.
The critical values obtained and recorded in the table above were obtained by making actual measurements of the quantities used during the construction process. It has been established that energy consumption by multilayered buildings is the highest and most energy-intensive. The typical value of energy consumption for a multilayered building per square meter of built-up area is 4.21 GJ per m2. However, for a two-storeyed building, the energy consumed is 2.92 GJ/m2, making it less costly by 30% compared with conventional methods (IEA 2010b, p.30).
However, with the use of alternative building materials, it has been shown that the energy consumed per building is 1.61 GJ/m2, using SMB filler substitutes. Based on research observations made by comparing both conventional and substitute materials in the context of energy savings resulting in a 50% reduction of energy (Easton 2000, p.3). The overall benefits are to conclude that substitute materials provide significant energy savings and environmental protection. Other benefits include simple techniques that can be understood and used by a majority of people with little technical skills, reduced energy consumption in the transportation and use of materials, provision for the use of decentralized production systems which accommodate and allows for the use of cheaper technologies, and the creation of local employment.
Future prospects
Future perspectives depend on a road map that has been built based on previous studies and research and development. The typical road map covers materials for constructing sustainable buildings.
The proposed energy perspective covers the reduction of CO2 emissions, based on the use and integration of low carbon technologies into the mainstream cement and concrete manufacturing processes. Estimated show a carbon reduction strategy includes carbon capture and storage of 19% of the carbon outputs, investment in renewable sources of energy, improving the power production efficiencies, implementing the end-use fuel-switching method which results in a 15% energy reduction and in the investment in safe nuclear energy (IEA. 2010b, p.5). Most of the current buildings were designed to last several years and are projected to be in use by 2050. Most of the developed nations rely on retrofitting the buildings, but the developing world will have to purchase energy-efficient technologies.
Cement and Concrete Technology
Cement accounts for the emission of large quantities of CO2 because of the energy embodiment. According to the Cement Sustainability Initiative (CSI), by integrating efficient engineering methods and cement manufacturing technologies, it is possible to reduce the emission of CO2 by half the current value by 2050. The critical path methods for the reduction of CO2 by 2050 include employing energy-efficient state-of-the-art technologies, improving thermal and electric efficiencies, and using other energy-efficient technologies.
To ensure a sustainable future there is a need to develop sustainable technologies, which factor environmental problems and issues and provide the platform for the reduction of CO2. The main objective of such technologies is to ensure social and environmental efficiency, which are quality drivers toward the achievement of sustainable development. In addition to that, technology should contribute to a healthy environment and encourage the efficient use of resources. One of the strategic approaches of reducing the greenhouse gas emissions, which is generated from the use and preparation of concrete and its ingredients, includes the intervention of governments, which have made legislation on how to control the emission of greenhouse gases. Another method of reducing greenhouse gas emissions for sustainable development is the use of new technologies for making cement, which is carbon-reducing cement.
Calcium carbonate-based cement is one of the cement products that can be made to substitute the high energy-consuming cement. The cement can be produced in a rotary kiln by use of aluminum and its compounds, which makes it less polluting to the environment. The results of this cement are that t has very high strength when compared with the normal Portland cement. The cement has a very good resistance to attack by chemicals and other corrosive chemicals, making it more suitable for use when compared with ordinary cement. The only problem with this type of cement is that it is expensive and causes an increase in slag, which might not be desirable.
Another type of cement that has been proposed for use is super sulfate cement. The cement is produced by grinding and mixing certain sulfates and burning them in the clinker. The resulting cement can be used for sustainable development and in areas that are vulnerable and exposed to acids and organic oils. Researchers have proposed the use of magnesium oxide-based cement as another substitute to the normal Portland cement Huovila & Koskela 1998). Magnesium oxide cement is produced from magnesium-related substances and has been shown to consume less than 30% of the energy consumed to produce the same amount of Portland cement. The production of low energy-consuming cement is critical for sustainable development. The cement that is produced from magnesium has proved to be of high strength and has been in use by the Chinese and other past civilizations for the construction of buildings and bridges. The advantage of this type of concrete is that it is of higher strength than Portland cement. This type of cement is in use in a wide variety of areas for construction purposes and has proved to be popular because of its high strength and low-cost Huovila & Koskela 1998).
It is possible to influence the strength of cement by adding new substances and chemicals such as magnesium oxide to cement. It has been provided that the cement produced from magnesium oxide uses a low amount of energy, which leads to low energy consumption and low emissions to the environment (Huovila & Koskela 1998).
Another form of cement that can be used for sustainable development is Sequestrated carbon cement. Research and development have led to the production of cement, which is similar to marine cement. Marine cement is the coral reef type of cement that is produced by the combination of magnesium and calcium in seawater with carbon dioxide, which is captured from the environment (Huovila & Koskela 1998). The result is a very strong type of cement, which is produced in the sea. The process can be mimicked using a process known as Calera cement. The Calera process is in the diagram below.
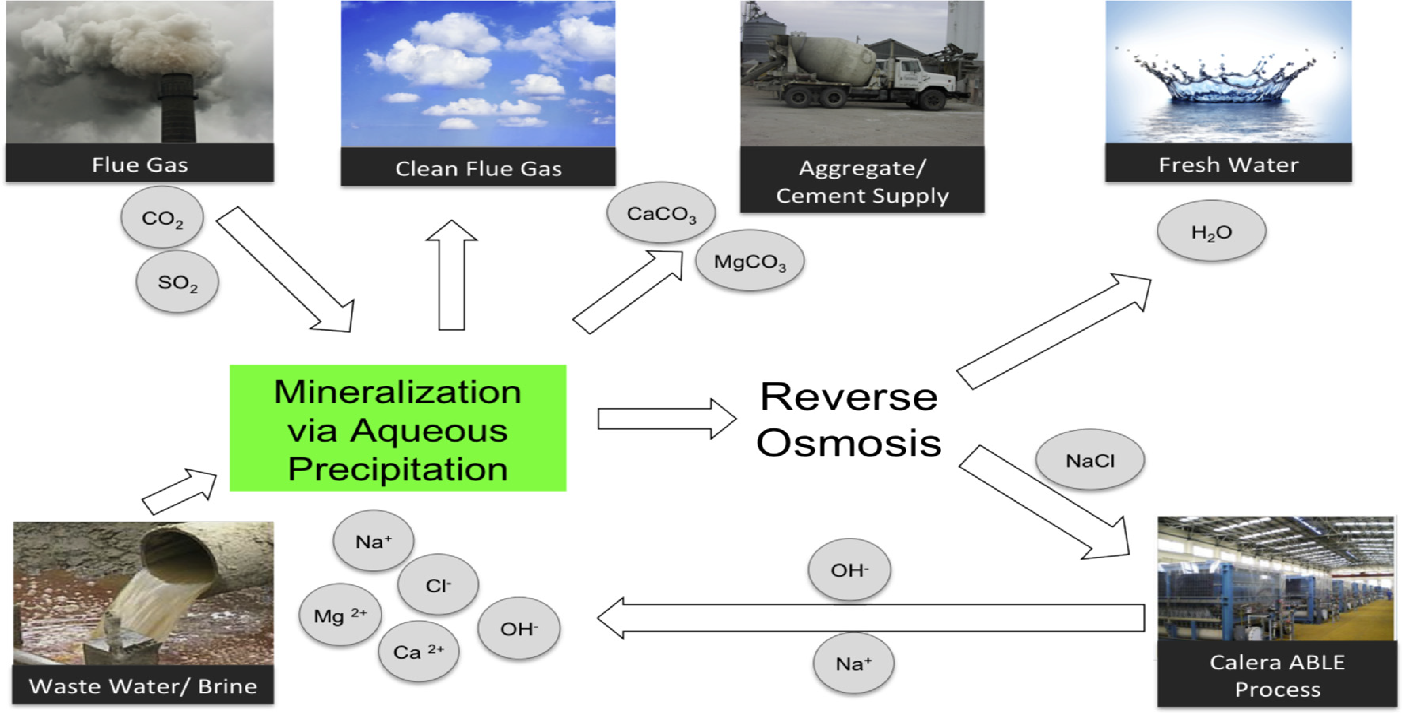
It can be seen from the above diagram that the flue gas is captured made into the mineral through aqueous precipitation. The next process is to convert the substances through reverse osmosis to produce clean water and NaCl, which undergoes the Calera process and the process, continues and becomes cyclic in nature.
Another approach is to use alternative fuels which are less carbon-intensive, less energy-intensive, and the use of biomass fuels as alternative sources of energy, and the use of substitute clinker with alternative cementitious alternatives.
Norton (1997,p.17) shows that it is possible for a reduction of emissions from the manufacture of cement as outlined in the general report by the United Nations World Commission on Environment and Development. The report urges all nations to set predetermined goals for the integration and use of energy-efficient technologies and other economic development goals based on the use of sustainable technologies. In each case, any economic model adopted by any country should reflect a sustainability approach to the use of and expansion of existing sources of energy. In addition, the exploitation of virgin raw materials and other secondary materials for the manufacture of cement and concrete should be based on sustainable methods and technologies. It is critical to understand that environmental sustainability is the core survival of mankind.
Other approaches that put the future into perspective are the use of nuclear-powered sources of energy. Despite the vulnerabilities such as environmental radiation and other forms of threats associated with nuclear power, nuclear sources of power are worth contemplating (Norton 1997, p. 5).
On the other hand, the future perspective holds that an assessment of the current concrete production methods and future energy-efficient methods which consume minimum resources to produce structurally efficient concrete be conducted to develop better technologies. It is important to perform continuous monitoring and to collect accurate data on the progressive reduction of CO2 emissions into the environment to assess the quality of technology in use and create methods to improve the efficiency if the technology. It is also critical to develop standards and specifications that integrate the aspects of the environment for the processing and production of concrete and concrete structures (Minke 2006, p.198).
Structural Stability
Minke (2006, p.98) shows that the intended service life of a building should be completed without any structural weaknesses detected before the completion period of the service life of the structure. It is important to note that the structural design methods have significantly improved in the recent past. The limit state design method is the most recent and widely accepted construction method. It is possible to determine the quality of materials used in the construction of such structures using the state limit design method. However, a new approach that is becoming increasingly popular is performance-based design. The performance-based design relies on compliance requirements for safety (Minke 2006, p.198).
According to IEA (2010a, p.45), facts show the need for an increased degree of safety in the design and development of structures. Increased safety leads to a decrease in the burden on the environment. In addition, the current and future trend is to improve the quality of materials used in the development of structures and to decrease the burden previous materials have caused on the environment. By investing in research, development, and innovation, better construction methods, materials, and improved structural and mechanical efficiencies lead to improved environmental safety (IEA. 2010b, p.23).
Energy issues
Research by Spiegel and Meadows (1999, 45) shows that energy-related issues account for the most critical components of sustainable processing and production of building materials. Here, environmental safety and suitability are critical issues of consideration (IEA-WBCS 2009, p.11). Examples include nuclear energy which accounts for 13.5% of the world energy production radioactive. Nuclear reactors are vulnerable to terrorist attacks and radioactive leakages that produce radioactive contamination because of nuclear disasters because of the meltdown of nuclear reactors. Some of the issues arising because of the use of nuclear power are radioactive contaminations and attacks from terrorists (IEA-WBCS 2009, p.12).
The Hannover Principle
It is possible to reach sustainable construction by judicious use of natural resources, which includes the use of recycled products and other waste materials to ensure sustainable environmental protection. The use of those material mentioned above has demonstrated that they lead to lower environmental impact, which leads to lower carbon and greenhouse gas emissions, a reduction in the use of concrete producing aggregates, which are extracted from quarries. Typically the use of recycled materials and other forms of aggregates underpins the goal of sustainable construction using low energy consuming and environmentally destroying materials.
Some suggestions have been put forward on different issues related to the production and use of materials for sustainable construction and one of the principles, which has received widespread acceptance, is the Hannover principle on designing for sustainability. Typically the principle provides” that the design is able to meet the needs of the present generation without compromising the needs of the people in the future generations” (Minke 2006, p.198). Typically, “the designer has to learn and be aware of the consequences of the design they make and its impact on the environment” (Minke 2006, p.198). If the design is not sustainable, it should be dropped and a new design created which factors issues of sustainable construction. Here, the design should factor issues of humanity and recognize the fact that there are long-term consequences associated with the design. The environment and man have to exist interdependently, which implies that man cannot destroy the environment to assume that he can continue to live. Neither can the environment exist without a man? Typically, the key components of the Hannover principle include respecting the relationship that exists between spirit and matter, which covers issues related to human settlement. The principle requires that the designs be of long-term value and the ability to recognize the interactive nature of different objects and their interdependence. The principle requires the designers to create objects of value and to ensure that the waste is eliminated in the design and construction of buildings. Other elements, which define the principle include the use of energy, which flows naturally, the use of responsive designs and the sharing of knowledge on new discoveries and designs for sustainable construction.
Demolition and reuse
Researchers in sustainable construction materials have established that building materials can be put to use once the lifetime of a structure has come to its end. The underlying reason is that millions of tons of waste materials are generated during the demolition of buildings all over the world. The embodied energy of creating new materials, which could cause the discharge of waste materials such as gases and toxic material can be avoided by reusing the material obtained from such demolitions. Typically, the reuse of concrete and other construction materials leads to the zero landfill solution, which could curb more use of energy to transport the waste materials and landfill problem, which has adverse impacts on the environment. Here, the concrete construction materials are designed properly, which makes them easy to reuse once a building has been demolished. It is also possible to crush concrete that has been recovered from a demolished building to make new aggregates and new concrete for construction purposes. Research shows that a concrete panel, which has been recycled has 30% to 40% more energy efficient than a similar concrete panel, which is made from new materials. The use of recycled concrete materials makes the cost of the concrete panel much cheaper compared with new concrete products.
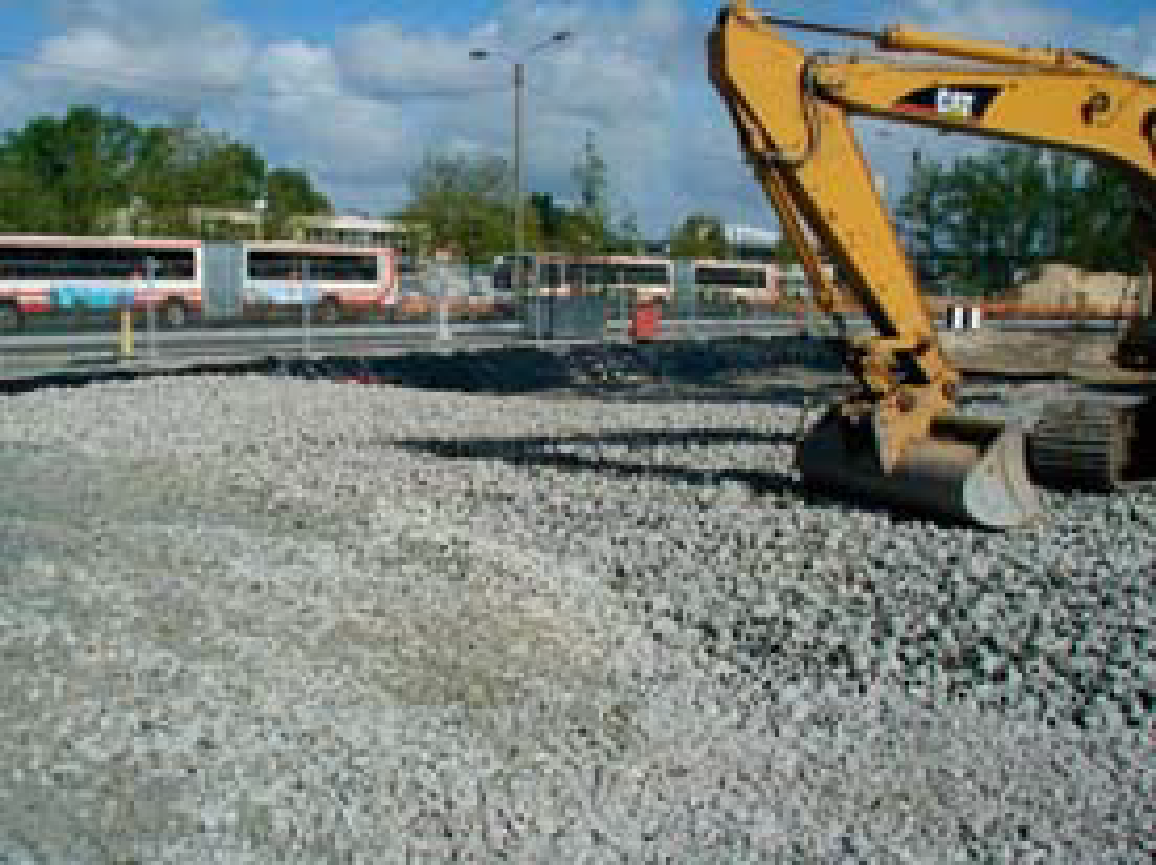
Material safety
It has been shown that the quality of materials used at the construction site has a lasting impact on the environment. Researchers recommend different strategies for improving the quality of building materials, which include the use of the minimal amount of materials or construction proposes. The best strategy is to reduce or to do away with structures, which require exorbitant use of construction materials to ensure minimal impact on the environment. A number of suggestions include the construction and use of high-performance buildings with water harvesting systems, which are compliant with the building codes of various countries. It has been suggested that using materials, which can be extracted from renewable materials provides a better system of reducing the impact such building materials have on the environment and provides the possibility of integrating the organic material processing cycle as a strategy to reduce the consumption of energy (Minke 2006).
Research Methodology
This paper was conducted based on the use of secondary sources of materials, which include journals and online sources of sustainable building materials and technologies in the construction and building industry (Hennink, Hutter& Bailey 2010). A significant number of research articles were analyzed based on the use of a qualitative research paradigm. The qualitative paradigm was based on the desktop literature review and analysis to accumulate the required information to address the research problem area (Taylor & Trujillo 2001). The rationale of using the qualitative paradigm was to enable the research to build a true picture of sustainable building materials and technologies in the construction and building industry, which are critical in solving the global environmental problems (Hennink, Hutter & Bailey 2010). The qualitative paradigm provided an in-depth understanding of a phenomenon or area of research and enabled the researchers to develop new perspectives and solutions to the problems in the area of research. The research method emphasizes on the use of the human instrument as the tool for conducting the research.
Findings
Research findings from the study provide a comprehensive source of information on different types of building materials and technologies, which are available for use in the construction and building industry, which are critical to achieving sustainability in the industry. The established comprehensive findings show that for sustainability to be achieved, the characteristics of materials for the sustainable site, which have a little ecological impact, reuse of existing materials, design for sustainability, and the need to design modular structures, which can be dismantled and reassembled can be used. Findings show that a wide range of options is available, which include the use of concrete for producing concrete and cement for sustainable development including the use of crushed glass. It is possible to use crushed glass, which is made into very fine particles to make substitutes for cement, which can account for up to 45% of the cement required for construction purposes.
Conclusion
In conclusion, researchers in the building and construction industry agree that the use of traditional and current construction materials, which undergo energy-consuming processes including cement, concrete, and steel translates to adverse impacts, which are evident in the environment because a lot of energy is used in the production of steel (Hammond & Jones 2008). The net effect is high energy embodied in steel, cement, and concrete in the materials. Different solutions have been suggested and one of them is to use synthetic fiber reinforcements, reuse waste products, adopt modular design methods to encourage reuse, and use synthetic fiber reinforcements to reinforce structures, which are not made from concrete. The quality of the reinforcements makes the synthetic fiber reinforcements applicable in different areas as a substitute where concrete could be used. Using synthetic fiber reinforcements concrete has been shown to improve the quality of concrete by preventing the concrete from cracking and being affected by environmental forces. In addition, synthetic fiber reinforcements can be made from recycled materials, which makes the material less costly and more cost-effective. Other suggestions include designing building products for reuse to encourage sustainability and investing in the development of technologies that promote reduced energy consumption and in other resources that consume low amounts of energy and less energy-consuming substitute technologies.
References
Anink, D, Boonstra, C & Mak, J 2004, Handbook of Sustainable Building: An Environmental Preference Method for Selection of Materials for Use in Construction and Refurbishment, James & James Publishers, New York.
Ayers, R U 2002, ‘Minimizing waste emissions from the built environment’, In Construction Ecology: Nature as the Basis for Green Building, vol. 1, no. 1, pp. 12-45.
Berge, B 2009, The Ecology of Building Materials, Architectural Press, New York.
Calkins, M 2012, The Sustainable Sites Handbook: A Complete Guide to the Principles, Strategies, and Best Practices for Sustainable Landscapes, Wiley, New York.
Calkins, M 2009, Materials for Sustainable Sites: A Complete Guide to the Evaluation, selection, and use of sustainable materials, Wiley, New York.
Ding, GK 2008, ‘Sustainable construction—the role of environmental assessment tools’; Journal of environmental management, vol. 86, no. 3, pp. 451-464
Easton, D. 2000. Rammed Earth. Alternative Construction: Contemporary Natural Building Methods, Wiley, New York.
Emmitt, S & Yeomans, DT 2008, Specifying Buildings: A Design Management Perspective, Elsevier Butterworth-Heinemann, New York.
Halliday, S 2008, Sustainable construction. Routledge, New York.
Hennink, M, Hutter, I & Bailey, 2010, Qualitative research methods. Sage, New York.
Hammond, GP & Jones, CI 2008, ‘Embodied energy and carbon in construction materials’, Proceedings of the Institution of Civil Engineers-Energy, vol. 16, no. 12, pp. 87-98.
Horvath, 2004, ‘Construction materials and the environment’, Annu. Rev. Environ. Resour., vol. 29, no. 2, pp. 181-204.
Huovila, P., & Koskela, L1998, ‘Contribution of the principles of lean construction to meet the challenges of sustainable development’, In 6th Annual Conference of the International Group for Lean Construction. Guaruja, São Paulo, Brazil, vol. 1, no. 2, pp. 13-15.
‘IEA’ 2010a. Energy Technology Perspectives: Scenarios & Strategies to 2050. International Energy Agency, Wiley, Paris.
‘IEA’ 2010b. Key World Energy Statistics. International Energy Agency, Paris.
‘IEA-WBCS’ 2009, Cement Technology Roadmap 2009: Carbon Emissions Reduction up to 2050, vol. 2, no. 2, pp. 23.
Jonkers, HM., Thijssen, A, Muyzer, G, Copuroglu, O & Schlangen, E2010, Application of bacteria as self-healing agent for the development of sustainable concrete. Ecological Engineering, vol. 36, no., 2, pp. 230-235.
Kibert, C. J, Sendzimir, J & Guy, G. B 2001, Construction Ecology: Nature as a basis for green buildings. Routledge, New York.
Taylor, BC & Trujillo, N 2001, Qualitative research methods. The new handbook of organizational communication: Advances in theory, research, and methods, 161-194.
Thompson, JW & Sorvig, K 2007, Sustainable landscape construction: a guide to green building outdoors. Island Press, New York
McGowan, MR & Kelsey K 2003, Interior Graphic Standards, John Wiley & Sons, New Jersey.
Mehta, PK & Burrows, RW 2001, Building durable structures in the 21st century. Concrete International, vol. 23, no. 3, pp. 57-63.
Mendler, S, William O & Mary A L 2006, The HOK Guidebook to Sustainable Design, Ho John Wiley & Sons, Hoboken New Jersey.
Minke, G. 2006. The technology of Sustainable Architecture, Birkhauser-Publishers for Architecture, Boston.
Norton, J 1997, Building with Earth: A Handbook. Intermediate Technology Publications, London.
Ortiz, O, Castells, F & Sonnemann, G 2009, ‘Sustainability in the construction industry: A review of recent developments based on LCA’, Construction and Building Materials, vol. 23, no. 1, pp. 28-39.
Peris Mora, E 2007, ‘Life cycle, sustainability and the transcendent quality of building materials’, Building and Environment, vol.3, no.42, pp. 1329-1334.
Spiegel, R & Meadows, D 1999, Green Building Materials: A Guide to Product Selection and Specification, Wiley, New York..
Williams, K & Dair, C 2007, ‘What is stopping sustainable building in England? Barriers experienced by stakeholders in delivering sustainable developments’, Sustainable development, vol. 15, no. 3, pp. 135-147.