Abstract
In science, when more than three or more chemical elements combine within a single system or vessel to form a component the resultant product is a compound that has in it some of the chemical properties of the individual elements. This type of reaction is referred to as a multicomponent reaction (MCR). The tendency of these compounds to exhibit some of the chemical properties of the formerly reactive elements gives a clear field for divergent molecules per unit time with the least synthetic, time, and energy consumption.
One multi-compound reaction consists of a series of stages of which later transformations are directly influenced by the products that were yielded in the previous stage. These reactions make up an essentially important synthetic channel since they facilitate open and quick access to the world of organic compounds that exhibit very large and dynamic patterns within which they substitute each other. MCRs reactions happen within a single vessel, they differ from multi-step synthesis reactions in that they occur in single pots.
Together with a thorough record screening, this approach opened up one of the most strategic developments through time by facilitating the discovery of drugs. This new phase opened the world to fast and efficient discoveries from the medicinal perspectives, harnessing the lead compounds within which happen to be very active biologically. Many of the minute organically composed compounds could perhaps be the best examples of prime candidates since the traditionally accepted peptides and oligonucleotides are limited to be used as biologically available treating agents.
Despite their myriad attributes to modern science and organic chemistry, in particular, they were mainly used in very small quantities compared to their usage in present times. The importance attached to these reactions was minimal and restricted, but five decades later they have had an insurmountable contribution to modern science thanks to the invention of the “high-throughput biological screening method.”
This has aroused interest and vigor in the research, and as such, all stakeholders from the research and academic fields have the sights keen to design and re-invent multicomponent applications to generate investments in heterocyclic compounds.
This mounting interest is motivated by the milestones gained and the potential the sector seemingly holds. Its therapeutic potential is so far unmatched and rigidity of the properly defined structures that define heterocyclic components has broadened its usability.
Introduction
Multi-component reactions (MCRs) play a significant role in combinatorial chemistry concerning molecular biology. It is among the latest innovations in the biological world that has seen chemical processes simplified in a manner that even minute compounds can be formed. MCRs come along with a couple of advantages that has seen previous methodologies relegated to the point that is regarded as obsolete.
The merits of this process have seen it attune to the environment since it is regarded as eco-friendly owing to the recyclable abilities of its by-products. With regards to medical chemistry, MCRs can synthesize a wide scope of drugs at ease owing to their structural flexibilities (Muarya 2008).
MCR is a unique chemical reaction that involves more than two chemicals to yield a product or more. This reaction unlike others constitutes a one-step operation (one-pot operation). This operation allows for simultaneous generation of products in a single vessel thus edging the previous methodologies in terms of economy.
MCRs constitute a wide range of reactions that include Ugi reactions, Passerini reactions, and Suzuki reactions among others. These reactions are named as per their building blocks (Pirrung 2005). For instance, the Ugi reaction is a reaction derived from α-aminoacyl amide, while the Passerini reaction is an isonitriles-based responsible for the formation of α-acyloxy carboxamides.
On the other hand, Suzuki reactions are aryl halide-based reactions that are enhanced by palladium catalyst. Since the inception of MCRs chemists did not relent in their quest for more efficient processes. Their craving for more efficient processes is owed to the continued evolving challenges that they have since been fighting. It, therefore, goes without saying that these processes have evolved to attune with present challenges. For instance, combinatorial chemistry which was previously used for triazine synthesis has been rendered obsolete owing to their generation of impure libraries (Muarya 2008).
This method saw to it the advent of the orthogonal solid-phase method which also did not impress much since; a Boc group formed and cleaved in the final stage limited its structural diversity. The recent process that overcame the demerits of these previous processes is known as the ‘Safety-Catch Method’ and, as the name suggests, it results in the generation of pure products (Coggiola et al 2005). This however is not a limit to innovations. As the nature of human problems continues to evolve so are the processes.
The literature that follows takes us through the background information of MCRs, the biological interests in MCRs, the evolving nature in MCRs synthesis of triazines and, the Suzuki reaction. Also, with regards to triazine synthesis, the paper includes some experiments performed in testing the merits of the current process.
Background information
The theory behind multicomponent reactions is not new to nature it has played a huge role in evolution so much so that adenine 10, the major element in the structure of the DNA and RNA came into being through the concentration of tentamolecules (5 major molecules) of HCN. HCN is also a major constituent in the prebiotic atmosphere (Appendino et al 2005). This is according to a reaction whose catalyst is NH3. Other nuclear bases have their origins tied to similar reactions that involve HCN and H2O.
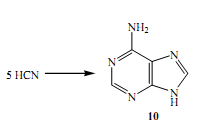
The initial interest and further invention of the modern evolution of multicomponent science was directed by Strecker in 1850 (Coggiola et al 2005).The most crucial step in the infamous strecker synthesis of amino acids comprises the formation of equivalent amino nitriles which are yielded from aldehydes 11, HCN and NH3 in a single reaction. The reactions that follow this up are mainly to hydrolyse the synthetically important results in the later reaction; the end product is amino acids.
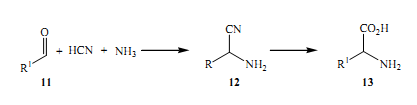
Further developments in this field is credited to Hantzsch, who around 1882 brought forth the idea of symmetrically replacing dihydropyridines where NH3 and aldehydes with two equal elements that summed up to B-ketoesters (Glan et al 2005).
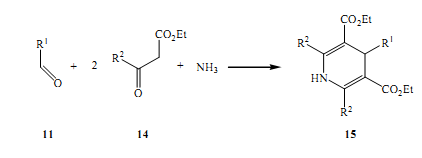
The other significant aspect of Hantzsch contribution to this branch of science was his discovery of pyrroles by mixing the primary amines to B-ketoesters 14 and ahalogenated (Zhang et al 1999)
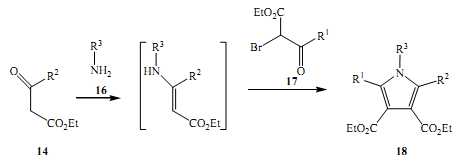
He conducted his first experiment in 1993, it is famously known as the Biginelli reaction. In this experiment, the synthesis of multicomponent elements replaced dihydropyrimidines 22, there was an acidic catalyst that was a cyclocondensed structure of B-ketoesters 19, and urea 21 and aldehydes 20, this composition of alddehydes were aromatic (Norris et al 2000).
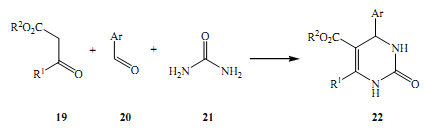
Immediately after its inception, the very first crucial application of multicomponent reactions was first applied in the synthesis of natural products. The first such experiment was done by Robinson, the aim of his first experiment was to synthesis an alkaloid, tropinone 25 from succinic dialdehyde, methylamine and salts (calcium) derived from acetonedicarboxylic acid (Muarya 2008).
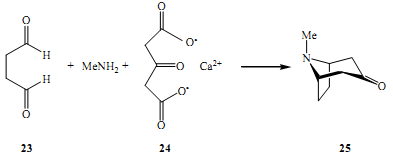
On the other hand, Passerini in 1921 conducted the first MCR which involved isocyanides. He used carboxylic acids, carboxyl compounds plus isocyanides (Newman & Craig 2007). This experiment yielded a-acyloxy carboxamides in a procedure that involved a single pot.
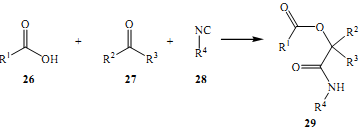
Sometime later at around 1934, another experiment still based on the principal of four component reactions was conducted by a pair of scientists, Bucherer and Bergs. Hey conducted a one pot reaction of elements such as hydrogen cyanide, aldehydes, NH3 plus CO2 (Xiang et al 2004). This experiment yielded hydantoins, a compound that could easily be transformed into a-amino acids through a simple process, hydrolysis (Pirrung & Sarma, 2004).
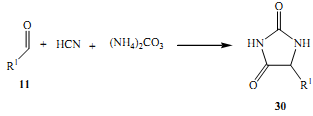
The other experiment that proves to be equally important was the Asinger experiment. It occurred in 1958 and involved a-Halogenated carbonyl compounds with sodium hydrogen sulphide to produce in situthiols. This further reacted with carbonyl compounds and ammonia to yield thiazolines.
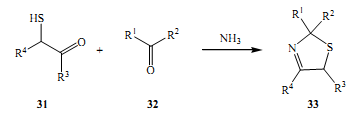
Ugi et al. founded in their experiments discovered one of the most important reactions over time in that it has been used time and time again among the multicomponent reactions (Doris & Kappe 2007). This was in 1959. It involved synthesis of a-acyclamino amides was achieved through the reaction of reacting several aldehydes with primary amines, isocyanides and carboxylic acids.
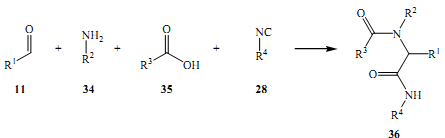
At around 1961, Gewald and his assistants illustrated the reaction of polysubstituted thiophenes with electrons, removing other elements like cyano, carboethoxy, and carboxamide in their 3-positions (Ugi 1962). Alkyl, aryl, cycloalkyl, and heteroaryl cluster in 4- and 5- positions.
The three main changes in this method are explained in theory, lending access to several 2- aminothiophenes (Dieltiens & Stevens 2007)Among them, the very well-designed and straightforward version that uses the single pot system, condensing aldehydes, ketones, or even 1, 3- dicarbonyl compounds. This requires the use of stimulated nitriles and sulfur with an amine.
All this happens at normal atmospheric conditions (room temperature). The most ideal solvents in this process are several; these include dioxane, methanol, ethanol, dimethylformamide, and ketones for example methyl ethyl ketone (Koinuma 2000). The amines that are often used are triethylamine, morpholine, or diethylamine.
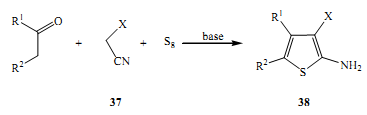
One of the largest and most critical branches of MCRs is multicomponent reactions involving isocyanides (Domling & Ugi 2000). The first one of these series as mentioned earlier was established in 1921 by Passerini. Their advantage over the wide array of multicomponent structures is their ability to interact with many secondary useful groups that are not involved in the initial stages of a multicomponent reaction (Cawse 2003).
Their biggest relevance to the complicated process is the premeditated prospect that these secondary groups can be used in other reactions that occur beyond this stage, this can occur through several processes.
Isonitriles are a group of compounds in a very important, though the secondary group. They have an abnormal valence makeup and also react differently. They have for a long time been used to represent stable organic compounds in their class (Cawse 2002). They also acted with a divalent carbon. Due to this fact, this group differs both structurally and functionally from all the other secondary groups (Bienayme et al 2000).
They are characterized by three properties that make them different, that is a-acidity, a-addition, and their tendency to easily form radicals (Aime et al 2002). Their characteristics vary further; they have a strange odor that is suggestive of artichoke fumes. Extended contact to these compounds of isocyanides “was associated with increasing the intensity of dreams at night” (Efskind & Undheim 2003)
This group of compounds was first produced in 1859 by Lieke who first mistook them to be nitriles. Gautier, another scientist Gautier revealed the isometric nature in their association with nitriles. This prompted Hoffman “to research and develop a novel technique by mixing the primary amines with potash to form chloroform” (Domling 2000).
The biological interest of multicomponent synthesis
With regards to molecular biology, one of the discoveries and developments that have revolutionized the way to go about different issues are MCRs (Enders, Grondal, Huettl 2007). These reactions have found their applications in different facets of the medical world. This includes medicinal chemistry, natural product synthesis, glycochemistry, and polyamines for diagnostic and therapy. MCRs evolved due to growing concerns over which the then methodology, combinatorial synthesis, was used to synthesize new medicine (Denmark & Fan 2005).
MCRs are flexible, unlike combinatorial synthesis which is rigid limiting structural diversity necessary for the increased rate at which new medicinal substances are synthesized. MCRs owe their structural diversity to their flexible functional heterocyclic building blocks that allow for versatility in bonding with different compounds yielding a spectrum of compounds (Dambruoso et al 2005). The advances in MCRs attune with the environment since they are eco-friendly, simple, and above all; safe for human beings.
Medicinal chemistry
On narrowing down to medicinal chemistry, the focus is skewed towards the design, creation, and assessment of the new compounds vital for biological as well as remedial interests. Medicinal chemistry uses two kinds of approaches to achieve these tasks: classic multistep synthesis and new tactics that include MCRs, combinatorial synthesis, and click chemistry (Feher & Schmidt 2003). Our interest though lies in the MCRs that utilize new functionalized groups (isocyanides, carbene, Ugi four-component reactions (U4CRs) to create a novel match for identified bioactive compounds (Dawane, et al 2009).
Isocyanides
The use of isocyanides in MCRs represents one of the ideal methodologies for medicinal synthesis in the name of a ‘Special kind of Tandem Reaction’ and, as the name suggests it occurs spontaneously yielding massively. Isocyanides-based MCRs (IMCRs) are unique vis a vis other MCRs (Donatella et al 2005). Its extraordinary nature is owed to the nature of its functional group which is known for its reactivity forming the subject for discussion that spans more than ten decades. The structure of isocyanide is represented as shown in figure 1 below.

This structure is understandably the reason why isocyanides are more stable relative to carbenes and carbon monoxides. Isocyanides occur either naturally or artificially. The former has been studied revealing a “strong antibiotic, insecticidal, fungicidal or antineoplastic effects combined with low toxicity for warm-blooded animals” (Ma et al 2006).
Artificial isocyanide is the brain child of Lieke, 1859, whom by fluke in an experimental set up in an attempt to synthesize nitriles from a reaction of alkyl iodides versus silver (I) cyanide produced isocyanide instead (Domling 2006). His experiment acted as a precursor for the advancement in the studies of synthesis and isomeric structures of the same in the later years (Ivar 2001).
The reactivity nature of isocyanides is however what stands out to be appreciated in the synthesis of analogues with respect to MCRs. This is characterized with respect to “three properties: the α-acidity, the α-addition and the easy formation of radicals” (Price 2007). For instance, in the synthesis of Kinase inhibitor (+)-K252a, the later characteristic comes in handy. Phenyl isocyanide undergoes radical rotation consequently yielding an inhibitor represented as is in the fig. 14 below (Xiang et al 2004).
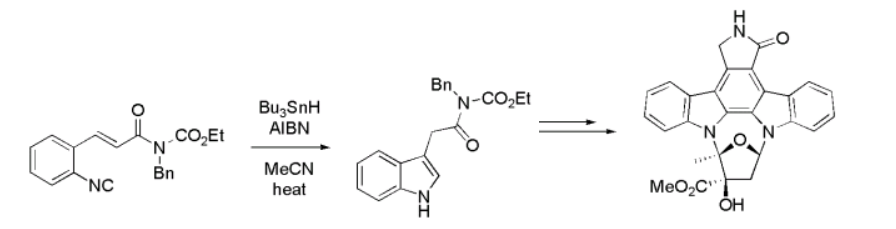
However, the most significant property of synthetically derived isocyanides stems from the α-addition characteristic that enables it to form α-adducts (Alexei et al 2006). This property is enhanced by the orientation of π-orbital and the nature of σ-orbital that are positioned on the same carbon: the former is positioned perpendicularly while the latter doesn’t bond.
Consequently, the compound exhibits dual properties of electrophilic and nucleophilic. With these effects, IMDRs can form a wide range of compounds that attune with the increased demand for medicinal substances (Armstrong et al 1996). To achieve α-acidity characteristics, the overall charge ought to be negative which is achieved by elimination of an ester group. The α-acidity property is vital when it comes to the “synthesis of heterocycles, α, β-unsaturated isocyanides and amino acids” (Xiang et al 2004).
Furthermore, isocyanides form the backbone in MCRs synthesis of skeletal structures of proteins that constitute peptidic (deposit) bonds thanks to Ugi and Passerini reactions. Additionally, they do find their applications in the building of biologically appropriate heterocycles that include “oxazoles, oxazolines, thiazoles, thiazolines, pyrroles, imidazoles and imidazolines” (Domling & Ugi 2000). In a nutshell, the advent of isocyanides has given medicinal chemists a chance for versatility concerning the generation of a wide variety of medicinal compounds.
Alzheimer’s Disease
With the advances in molecular chemistry, MCRs have seen the development of diverse libraries vital in solving many biological issues. One of the advances in medicinal chemistry has seen the development of new heterocycles that contain amidine. These libraries produce exceptional interactive patterns between “β-Site Amyloid Cleaving Enzyme (BACE) catalytic diad and a weak NMR screening hit” (Moreira et al 2006).
This unique reaction attunes well with reversing or even halting the trend of otherwise fatal Alzheimer’s disease (AD). AD, which is understandably a neurodegenerative illness, accounts for a total of 8 million patients in the U.S. alone. Once infected the victim has less than a decade to live (Moreira et al 2006).
AD is characterized by the formation of extracellular amyloid that contains amyloid β peptide composed of 42 protein building blocks (Aβ42) and, an intracellular mesh of hyper-phosphorylated protein. Aβ42 is embedded in the brain causing a lot of damage to the brain organ. The initial stage of the disease is characterized by an increased rate of Aβ peptide formation thanks to the mutating nature of amyloid precursor protein (APP).
Patients with Down’s syndrome are understandably more vulnerable to the onset of AD than their healthy counterparts; their extra gene has APP (Moreira et al 2006). Aβ peptides with amino acids building blocks that fall between 37 and 42 are distinct from each other as at C-terminus.
These peptides are produced in minute quantities as products of metabolism of a “membrane-bound APP via two consecutive cleavages, first by β-site APP cleaving enzyme (BACE-1, also known as β-secretase or memapsin-2), followed by γ-secretase” (Moreira et al 2006). Studies done on mice have shown that BACE-1 KO is an effective precursor vital in the elimination of Aβ peptide generation and consequently halting plaque formation significantly in the memory lapse.
These studies acted as a green light to the synthesis of BACE inhibitors to remedy AD disease. Studies done on BACE-1 have shown that thiourea 3 represents an active site of the inhibitor. A compound of lead (Fig 15) composed of a 2-amino-dihydropyrimidinone ring is reported to have a biphenyl compound at carbon number 6 that acted as a precursor for the development of the inhibitor.
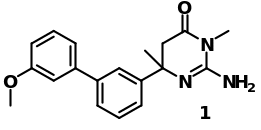
Natural products synthesis
In an effort aimed at supplementing the available naturally occurring compounds for convenience coupled with increasing demand for the same, the man went a notch higher by trying to mimic these compounds. However, the naturally occurring compounds eclipse artificial compounds with regards to efficiency; posing a continued challenge to man owing to the ever-evolving nature of natural compounds ever since (Khersonky et al 2003)Wohler, the scientist behind the manufacture of the first organic compound; urea, paved way for advances in technologies aimed at imitating naturally occurring compounds (Jiang et al 2010).
Many approaches have been employed though by chemists the world over in their quest in trying to emulate these naturally occurring compounds. One such tactic used is MCRs which are still underexploited concerning this field. MCRs are alluring owing to their mode of reactions: are “highly step-economical” (Madare 2008).
All said and done, man has considerably developed several compounds by the MCRs approaches which come along with advantages of simplicity, diversity as well as quantitatively. The literature that follows takes us through some of the compounds developed by man in an attempt to mimic naturally occurring compounds (Kumar 2008).
Prostanoids
Prostanoids have held scientists the world over hostage owing to their intricacy in their makeup their central task as local hormones in mammals and some animals notwithstanding (Jiang et al 2009). They are known for their vital remedial capabilities in these organisms. However, the quantities manufactured naturally from their sources are diminutive.
This has created lots of interest from molecular biologists keen to develop artificial hormonal substances synonymous with prostanoids to supplement the natural compounds. For more than twenty years now Noyori’s three-component strategy, MCRs have been used in the large-scale manufacture of this compound. This employs “tandem conjugate addition on cyclic enone followed by electrophilic trapping of resulting enolate” (Avalos et al 2006)This method was borne courtesy of advanced efforts in trying to solve problems that resulted from the previous methodology which resulted in minimal yields.
Terpenoids
These are naturally occurring compounds that find their applications in food industries used as aroma contributors. This compound is a derivative of Penta-carbon isoprene units that have been amassed and tailored to yield this compound. Research interests in terpenoids were catapulted by the assumption that their structure can easily be accessed through effortless acyclic polyene precursors (Ghetta et al 2005).
The synthesis of this compound is well elaborated by Corey and co-workers who utilized the idea of MCRs that allowed for “a three-component carbonyl addition as well as alkylation reaction sequence” (Bentabed-Ababsa et al 2008). Unique to this process is the Brook arrangement, a process that allows for the relocation of saline groups to oxygen from carbon.
This process is characterized by the addition of alkenyllithium intermediates that elicit a tandem Brook rearrangement consequently yielding the compound (Rossen et al 1998). Advances in the later years have seen the development of several compounds that include acetylcholinesterase inhibitors among others.
Many more other compounds have been developed courtesy of MCRs that includes polyenes and policies, oxacyclic products, and piperidines just to mention but a few. This has had a positive impact on molecular biology that has seen man cope with the challenges presented to him by nature.
Polyamines for diagnostic and therapy
Concerning diagnostic and therapy, the focus shifts to synthetic organic chemistry. Polyamines are known organic compounds that are extensively applied in biological systems vital for their critical roles. Their crucial roles are as a result of their “basic and metal chelating properties” (Gedey & Fulop 2002).
Artificially functionalized polyazacloalkanes which form an integral part of polyamines represent uniquely a class of metal chelating agents of a kind. They can form stable and multifaceted compounds with a spectrum of compounds composed of heavy metals (De Paolis et al 2008). Metal chelates are significant in magnetic resonance imaging (MRI) and radiopharmaceuticals vital for analysis and remedy of tumors. The applications of metal chilates in the duo are facilitated by their abilities as contrast enhancing agents (CAs).
The new emerging challenge that medics continue to grapple with though is generating new chelates that have the abilities to target specific organs and tissues (Bonnaterre, Bois-choussy & Zhu 2006). One of the tactics that is in the pipeline now as medics try to come to the bottom of this is by generating metal chilates that targets biological carriers.
These carriers are known as biofunctional chelating agent (BFCA). BFCAs have the abilities to bond with a wide scope of groups that includes “ketones or aldehydes, amine, carboxyls, isothiocyanates, maleimides, alkynes and vinylsulfone” (Banfi & Riva 2005). BFCAs abilities to bond with these compounds constitute MCRs that represents a step- economical process. In particular, it utilizes U4CRs.
Previous methods of synthesis of Triazine diones and Suzuki
Previous methodologies as regards MCRs cannot be undermined since they represent the basis behind the contemporary methods of syntheses. In order to comprehend the concepts behind novel methodologies with respect to triazine diones and Suzuki reactions, an in-depth analysis of the forerunning principles come in handy. They act as ‘a yard stick’ towards advancements in MCRs (Miyaura & Suzuki 1979).
Prior to the discovery of microwave assisted reactions, the driving force behind chemical reactions relied heavily on the use of Bunsen burner as a source of energy. In the later years as chemical reactions underwent revolution “heating mantels, hot water baths, and oil baths came into the centre stage of chemical reactions” (Miyaura, Yamada & Suziki 1979).
These energy sources identified themselves with the then chemical reactions which are becoming obsolete. The reasons behind the phasing out of the previous methodologies are attributed to the slow rate at which products are made, and low volumes of the products (Pirrung 2005). In addition, the previous methodologies are not eco friendly and, are cumbersome once executed (Nicholas & Marco 2003).
To surmount these impediments MCRs was discovered simplifying reaction while yielding pure compounds necessary for different applications. Among the common MCRs reactions is the Suzuki reaction which owes its origin to Akira Suzuki. Triazine diones represents a product of MCRs that is vital in medicinal chemistry. These previous methods have however undergone modifications in an effort to attune with the ever evolving nature of human problems (Nicholas & Smith 2006).
The perception of MCRs as applied in molecular biology is not unfamiliar to man. As regards evolution, it is understood that adenine, an important compound that forms an integral part of the DNA and RNA, is a product of condensation process. This process represents one of the previous MCRs methods used in the building of compounds. In this case, five molecules (HCN) catalyzed by ammonia gave rise to this compound as shown in the figure 15 below (Sambasivarao, Kikali, Dhurke 2002). It is understood that other nuclei bases have been synthesized through the same procedure.
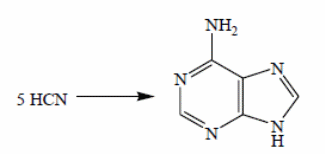
Combinatorial chemistry is among the MCRs ventures pursued by man in the synthesis of triazine diones in the recent past. Its application in medicinal chemistry accounts for a greater contribution in terms of discoveries of new drugs (Simons 1980). The use of triazine in combinatorial store scaffold is attributed to its properties that allows for versatility. Triazine, which has a structure synonymous to purine portrays a wide scope of biological activity (Short & Mjalli 1997).
An extensive documentation of both solid-phase and solution exist today. The drawback of this process however has a bearing on the process path. The process path is represents a stepwise animation process that is known for the accrual of by-products tainting products quality.
Multicomponent synthesis
Triazine diones
Carbon-based reactions cleave in many different forms that are more than a billion times. These reactions provide for a wide chemical space that allows for the formation of a variety of molecules (Sun et al 2000). To date, only a limited number of these organic compounds have been exploited.
An effective one-pot artificial procedure towards the synthesis of triazinane diones that still remains underexploited merges isocyanates, nitriles, phosponates and aldehydes. This reaction, a four-component reaction (4-CR), gives the organic chemists a host of advantages that allows for the diversification of libraries as well as providing them with optional paths vital for the synthesis of triazinane dione libraries (De meijera, von Zezschwitz, Brase 2005)
This reaction once executed allows for the formation of a functional group 3,4-dihydropyrimidine-2-ones (DHPMs, 5) as a result of cycloaddition reaction with isocyanates 4, courtesy of an electron-extracting substituent. This is represented in the scheme 16 below.
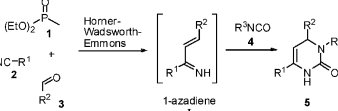
The reaction is executed through 1-azadienes that may transform to aza-Diels-Alder (aza-DA) cyclic addition with isocyanates. This reaction has a limited activity towards dienophiles consequently limiting the reaction between aza-DA and 1-azadienes. This has a negative effect with respect to scaffolds synthesis. Initial studies revealed the formation of noncyclized 6 together with triazinane diones 7a as shown in the scheme 17 below.
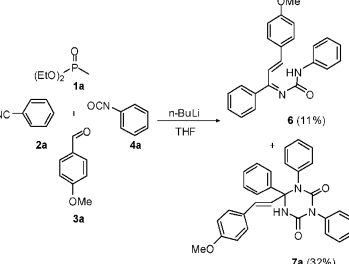
The classes of compounds represented by the compound 7 are under exploited prompting interests in organic chemistry with regards to triazinane synthesis (Domling & Ugi 2000). The reaction path through which 7a is formed is represented in the scheme 18 below.
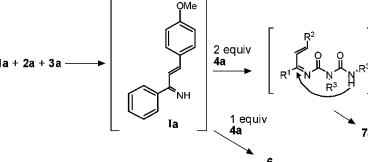
The partially isolated molecule 7a gives chemists an opportunity to maximize on the scope of MCR pathway enhancing for the synthesis of several libraries. Some of these libraries are shown in the figure 18 below.
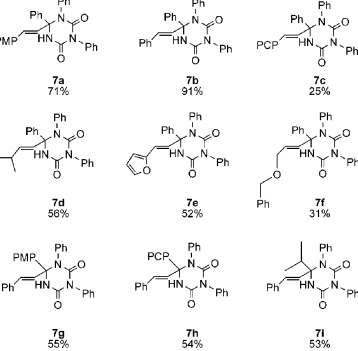
MCR always occurs efficiently when one either uses untainted nitriles or isocyanates.
Suzuki reactions
Libraries that result from fluorous methods are what constitute the Suzuki reactions. Professor Wei Zhang has done a lot of literature on studies concerning fluorous processes eclipsing other scientists in the same area. This literature does majorly cover MCRs as the basic method in the synthesis of these libraries. It is understood that by “merging more than three molecules MCRs can form an enormous molecule in a solo step” (Barder et al 2005).
Moreover, this process achieves this within the shortest time possible resulting in the formation of multifaceted products. This has overshadowed many other processing methods making MCRs the most embraced of the existing processes. MCRs approach attune well with fluorous tagging: “one of the reagents acts as limiting component while the others are utilized maximally to ensure for the completion of the reaction” (Gian et al 2005).
The function of fluorous is to “tag the limiting element which then uses the fluorous solid-phase extraction (FSPE) to fragment a product” (De paolis, Teixeira & Tarot 2009)In their recent advances in MCRs, Professor Zhang et al ventured in fluorous tags using microwave synthesis (Tempest 2005).
This method though is dissimilar to Yang et al who ultimately came up with the same product but on a different approach. Unlike Yang et al, Professor Zhang employed the use of fluorous tag which gives him an edge over the former: it enhances the purification of the intermediates coupled with an increased level in multiplicity (Xiang 1995).
The reaction commences with three component MCRs to synthesize tetrahydroquinoline. This happens to owe to the presence of Lewis catalyst and irradiation by microwave. The resulting component was then “reacted with a submarine under acid catalysis and microwave heating to provide the bridged hemiaminal” (D’souza & Mueller 2007).
The resulting fluorous sulfonate in hemiaminal forms an integral part in Suzuki coupling giving rise to an assortment (eight) of the final products. FSPE is known to minimize wastage of solvents: perfluorosulfonic acid “by-product in the Suzuki reaction can be recovered in the fluorous wash, and ultimately being converted to sulfonyl chloride or fluoride, and reused” (Kaim et al 2006).
Suzuki Coupling
Palladium-catalyzed cross combination in a reaction between organoboronic acid and halide represents the pioneering reaction of the Suzuki Coupling. The final reaction results in the formation of (E)-6-(2-(6,6-dimethylbicyclo[3.1.1]hept-2-en-3-yl)vinyl)-1,3-diphenyl-6-vinyl-1,3,5-triazinane-2,4-Dione represented in the figure 19 below.
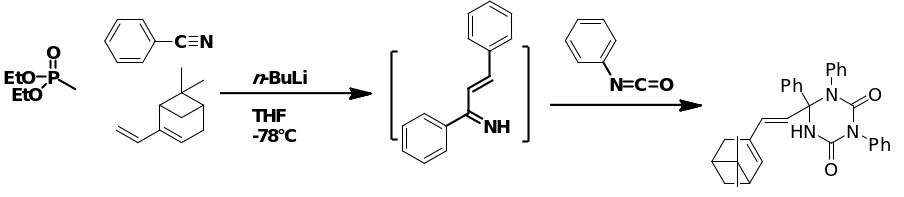
Advances in the catalyst and processing methods in the recent years have enhanced their applications immensely, extending the range of reaction components beyond aryls to encompass alkenyls, alkyls and alkynyls. Boronic acid alternatives include organoboranes and trifluroborates esters. Furthermore, “pseudohalides e.g. triflates may alternatively be used as coupling counterparts” (Ugi & Heck 2001).
In Suzuki coupling, [BMIM] BF4 is injected into the reaction. The catalyst in the reaction, [Pb (PPh3)2(Ar)] [X], is a consequence of an oxidative reaction that resulted from the transformation from aryl halide to [Pb (PPh3)2(Ar)]. The “hydrophobic ionic liquid does not compete with the unsaturated organic substrate for electrophilic active metal centre” (Armstrong et al 1996).
Vigorous air-stable systems as regards Suzuki coupling reactions with respect to bromobenzene using tolylboronic acid at a temperature of 110o C in Na2CO3 is achieved by “the addition of a substituted imidazole and (CH3CN)2PbCl2 as palladium(II) source to ionic liquids” (Armstrong et al 1996). Heating in the reaction came in handy in the generation of an absolutely colorless catalytic liquid long before the commencement of the reaction. Heating initiates the reaction vital in massive yields.
The reactivities of most stable solutions have a bearing on both cationic and ionic nature of the ionic solution. To improve there performance, both 1-phenylimidazole and 1-methylbenzimidazole is used resulting in the formation of the most active catalyst in the name of [BMIM]BF4. The rate “of hydrogenation of both aromatics and unsaturated and unsaturated hydrocarbons with a catalyst in ionic liquids is often controlled by hydrogen solubility in the liquids” (Armstrong et al 1996).
During the hydrogenation process of triazine dione, the compound (E)-6-(2-(biphenyl-3-yl) vinyl)-1, 3, 6-triphenyl-1, 3, 5-triazinane-2, 4-Dione undergoes transformation (through substitution process) forming intermediate compounds that consequently yield 6-(2-(2′-methoxybiphenyl-3-yl) ethyl)-1, 3, 6-triphenyl-1, 3, 5-triazinane-2, 4-Dione (Fig 20 below).
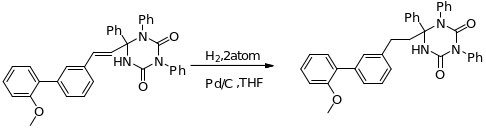
With regards to “the mechanism of Suzuki Coupling unlike Stille Coupling the boronic acid ought to be excited courtesy of a basic element” (Nicholas & Smith 2006). Excited and hence activated boron boosts polarization of organic ligand together with transmetallation. If the initiating “materials are replaced with base labile groups e.g. esters, powdered KF effects this activation while leaving base labile groups unaffected” (Nicholas & Smith 2006).
Figure 20 below shows how this is achieved. The uniqueness of boronic constituted Suzuki Coupling coupled with its low toxicity levels has created lots of interests resulting in the continued developments in the field. This is evident as reports regarding the same are continually being processed day in day out.
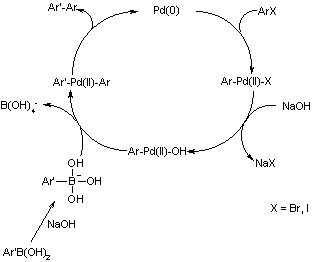
Experiment
To show the resourcefulness of MCRs methodologies a set of experiments were set up to synthesize acylated libraries.
Combination of thiophenol Resin with Monosubstituted Triazine dione
A range of monosubstituted triazines (20mg, 96.6µmol) was added to a suspension of PS-thiophenol resin (20mg, 25.6µmol) in tetrahydrofuran (THF) (2ml) to which was followed by the addition of N/_N-diisopropylethylamine (DIEA) (40µl, 0.31 mmol). The mixture was then subjected to a temperature of 650C for 3 hours. PE frit cartridge was used to sieve solvents and surplus reagents and; later followed washing with DMF, DCM, and MeOH (3 Ml *3) in that order. This was ultimately followed by washing with DCM (3 ml) and finally drying under nitrogen gas (Jackson & Haddad 1990).
Second chlorine replacement
A range of amines (NH2R2) (0.2 mmol) was added to a suspension of resin in N-methyl-2-pyrrolidinone (NMP) (1ml) and n-butanol (1 ml). DIEA (40 µL, 0.31mmol) was later on added before subjecting it to a temperature of 120 °C for 3 hours. This was finally followed by washing just like the previous experimental setup. However, drying was not performed (Jackson & Haddad 1990).
Launching of the final replacement site with oxidation of Thioether to Sulfone
An aqueous solution of sodium hydroxide (1 N NaOH) (40 µL) was added to a solution of m-CPBA constituting 3-chlorobenzene acid (70-75%, 56 mg, 0.23 mmol) in 1, 4-dioxane (1.8 mL). This mixture was then added to a resin and, this was gently shaken for 4 hours at ambient temperature. The solution was then sieved through a PET frit cartridge, after which it was washed with 1, 4-dioxane (3 mL *3) and DCM (3 mL *3). The final washing was done by DCM (3 mL) before drying in nitrogen gas (Gjerde & Fritz 2000).
Displacement of product from excited Sulfone Support by Nucleophile
PS-DIEA resin (2 mg) and nucleophile (12.8 µmol) were added to a suspension of excited sulfone resin that was in anhydrous acetonitrile (2 mL). The solution was then cleansed with nitrogen gas before placing it in a heating block at a temperature of 90 °C for 8 hours (Yongxiang et al 2007). DCM (1 Ml*3) was then used to wash the resin after filtering through a PE frit cartridge. The reagent from the eluent was then removed in a vacuum and, the products were later on dried under nitrogen gas (Yongxiang et al 2007).
Acyl Derivatives
2 MLS of 10% TFA suspended in DCM was used as a reagent in dissolving 40 mg of compound 28 shown in figure 17 above. This was then followed by washing of the filtered Boc-removed resin (using DMF, MeOH, and DCM consecutively). Drying using nitrogen gas then followed. 5 mg of resin was then suspended in NMP (1mL) and five matching of pyridine. To the solution, acyl chlorides were then added and then stirred for 3 hours at ambient temperature. The resin was then filtered before washing in a procedure synonymous with the first experiment (Yongxiang et al 2007)
Summary
MCRs defined as a reaction involving more than two chemical elements has seen to the development of novel compounds in an economical and yet efficient process. These reactions bear different names owing to the nature of the reaction and the building blocks. For instance, while Suzuki reactions are aryl halide-based reactions, Ugi reaction is α-aminoacyl amide-based. MCRs have undergone an evolution over time with the changing nature of human problems.
MCRs reactions constitute a wide variety of reactions. As regards triazine synthesis, the process has greatly changed: from combinatorial synthesis to orthogonal safety catch methodology that has seen for the generation of novel analogs necessitating biological processes. Similarly, the Suzuki reaction has undergone a massive change with the contemporary process which is microwave-assisted has aided in the generation of new compounds. These compounds include alkenyls, alkyls and alkynyls (Ugi & Heck 2001).
Conclusion
PS-Thiophenol in the first experiment played a significant role in affixing of the mono-substituted triazines. The second chlorine phase experiment was performed at a temperature of 1200C in a company of N/N-diisopropylethylamine.
This temperature resulted in the best performance of sulfur solid support in the displacement process. Sulfone’s excitement is owed to oxidation properties of thioether courtesy of m-CPBA. The conditions however should be monitored by the presence of NAOH. This helps in maintains the pH at around 4, hindering the removal of acid oriented groups from the structure. Nucleophile played a vital role in substituiting the already excited sulfone solid support to enhance the elimination of trisubstituted triazines. Anhydrous acetonitrile aided in limiting of the amount of hydrolyzed product.
The products were monitored using liquid chromatography (LC) and, the results revealed an absence of impurities from these compounds. In an effort to analyze the degree to which the process is limited to with regards to the last phase, activated sulfone that encompassed amine, aniline, and alcohol nucleophiles were used to attack the solid-support.
Their choices were owed to their wide scope of reactivity. Surprisingly, analines which are perceived to be having low nucleophilicities resulted in the production of significantly big amounts of products. Amines gave the most pure of all final products. In a nutshell, safety-catch method owes its success to its property (not acid-liable) that allows it to synthesize “acid sensitive triazine library products” (Bienayme 2000).
References
Aime, S, Cavallotti, C, Gianolio, E & Giovenzana, G, B 2002, Mannich Reaction as a New Route to Pyridine-Based Polyaminocarboxylic Ligands, Insubria University Press, Varese.
Alexei, V, K, Mikhail, K, Irina, K & Alexandre, V 2006, Tricyclic Products of Tandem Ugi 4CC/Intramolecular Diels-Alder Reaction, Journal of Organic Chemestry, vol. 71 no. 25, pp 9544 – 9547.
Appendino, G, Daddario, N, Minassi, A, Schiano, M, De Petrocellis, L & Di, M, V 2005, The Taming of Capsaicin. Reversal of Vanilloid Activity of N-Acylvanillamines by Aromatic Iodination, MacMurray, Denver.
Armstrong, R, W, Combs, A, P, Tempest, P, A, Brown, S, D, Keating, T, A 1996, Multicomponent Chemical reactions, Scribner’s, New York.
Avalos, M, Babiano, R, Cintas, P, Hursthouse, B, Jimenez, L, Lerma, E, Light, E & Palacios, C 2006, A one-pot domino reaction in constructing isoorotate bases and their nucleosides. Tetrahedron Letters, Vol. 47 no.12.
Banfi, L & Riva, R 2005, The Passerini Reaction. Journal of Organic Reactions, Vol. 65 no. 4, pp. 34.
Barder, T, Walker, D, S, Martinelli, J, R & Stephen, L 2005, Catalysts for Suzuki-Miyaura Coupling Processes: Scope and Studies of the Effect of Ligand Structure, Pergamon, Oxford.
Bentabed-Ababsa, G, Derdour, A, Roisnel, T, Saez, A, Domingo, R & Mongin, F 2008, Polar [3+2] cycloaddition of ketones with electrophilically activated carbonyl ylides. Synthesis of spirocyclic dioxolane indolinones. Organic & Biomolecular Chemistry, Vol. 6 no.17, pp. 3144-3157.
Bienayme, H, Hulme, C, Oddon, G & Schmitt, P 2000, “Maximizing synthetic efficiency: Multi-component transformations lead the way”, European Journal of Chemestry, vol. 8 no.16, pp. 3321–3329.
Bonnaterre, F, Bois-Choussy, M & Zhu, J 2006, ‘Rapid Access to Oxindoles by the Combined Use of an Ugi Four-Component Reaction and a Microwave-Assisted Intramolecular Buchwald-Hartwig Amidation Reactio’, Journal of Organic Chemestry vol.19, pp 4351 – 4354.
Cawse, J, N 2002, Experimental Design for Combinatorial and High Throughput Materials Development, John Wiley and Sons, London.
Cawse, J, N 2003, ‘Progress in Organic Coatings’, Journal of Applied Chemistry, vol. 47 no. 2, pp. 128-135.
Coggiola, B, Pagliai, F, Allegrone, G, Genazzani, A, & Tron, G 2005, Synthesis and biological activity of mustard derivatives of combretastatins, Bioorganic Medical Chemistry Letters. Vol. 15 no. 15, pp. 3551-3554.
Dambruoso, P, Bassarello, C, Bifulco, G, Appendino, G, Battaglia, A, Fontana, G & Gomez-Paloma, L 2005, Advances in the Universal NMR Database Approach. 2’-Substituted Taxanes as Probes for an Improved Protocol of Diastereomieric Differentiation, Organic Letters, no. 7, pp.983-986.
De Meijere, A, von Zezschwitz, P & Brase, S 2005, The virtue of palladium-catalyzed domino reactions – diverse oligocyclizations of acyclic 2-bromoenynes and 2-bromoenediynes. Accounts of Chemical Research, Vol.38, no. 5, pp. 413-422.
De Paolis, O, Baffoe, J, Landge, M & Torok, B 2008, Multicomponent domino cyclization-oxidative aromatization on a bifunctional Pd/C/K-10 catalyst: an environmentally benign approach toward the synthesis of pyridines, Organic Synthesis, no.21, pp. 3423-3428,
De Paolis, O, Teixeira, L & Torok, B 2009, Synthesis of quinolines by a solid acid-catalyzed microwave-assisted domino cyclization-aromatization approach.Tetrahedron Letters, Vol. 50 no. 24, pp. 2939-2942.
Denmark, E, S & Fan, Y 2005, “Catalytic, Enantioselective α-Additions of Isocyanides: Lewis Base Catalyzed Passerini-Type Reactions”, Journal of Organic Chemistry, nov.70 no 24, pp. 9667–9676.
Dieltiens, N & Stevens, C, V 2007, Metal-free entry to phosphonylated isoindoles by cascade of 5-exo-dig cyclization, a [1,3]-alkyl shift, and aromatization und microwave heating. Organic Letters, Vol.9, no. 3, pp. 465-468.
Dömling, A & Ugi, I 2000, “Multicomponent Reactions with Isocyanides”, European Journal of Chemestry, vol. 8 no.16, pp. 3325–3345.
Domling, A & Ugi, I 2000, Multicomponent reactions with isocyanides. Angewandte Chemie, International Edition, Vol.39 no.18, pp. 3168-3210.
Domling, A 2000, “The discovery of new isocyanide-based multi-component reactions”, Current Opinion in Chemical Biology, no. 4, pp. 318-323.
Domling, A 2006, Recent developments in isocyanide based multicomponent reactions applied chemistry. Chemical Reviews, Vol. 106, no. 1, pp. 17-89.
Donatella, B, Gian, T, Antonella, D, Roberta, F, Alberto, G, Elena, P, Gianni, M & Amedeo, L 2005, New Potential Uroselective NO-Donor α1-Antagonists, Journal of Medicinal Chemistry, vol. 46 no.17, pp 3762–3765.
Doris, D & Kappe, O 2007, “Microwave-Assisted Synthesis in Water as Solvent.” Chemical Reviews, vol. 107 no. 6, pp. 2563-591. Web.
D’Souza, D, M & Mueller, T, J, J 2007, Multi-component syntheses of heterocycles transition-metal catalysis. Chemical Society Reviews, Vol.36, No.7, 1095-1108.
D’Souza, D,M, Liao, W, W, Rominger, F, Mueller, T, J, J 2008, Dichotomies microwave-assisted propargyl-isomerization-Claisen domino sequences depende on base strengths. Organic & Biomolecular Chemistry, Vol. 6, no. 3, pp. 532-539.
Efskind, J & Undheim, K 2003, High temperature microwave-accelerated ruthenium catalyzed domino RCM reactions. Tetrahedron Letters, vol. 44, no.14, pp. 2837-283.
Enders, D, Grondal, C & Huettl, M, R, M 2007, Asymmetric organocatalytic domi reactions. Angewandte Chemie, International Edition, vol. 46, no.10, pp. 1570-1581.
Feher, M & Schmidt, J,M 2003, “Property Distributions: Differences between Drugs, Natural Products, and Molecules from Combinatorial Chemistry”, Journal of Chemical Reactions, vol. 43 no. 218, 45-50.
Gedey, S & Fülöp, F, 2002’,”Liquid-Phase Combinatorial Synthesis of Alicyclic β-Lactams via Ugi Four-Component Reaction”, Journal of organic chemistry, vol.4, pp. 1967–1969.
Ghetta A, Prosperi, D, Mantegazza, F, Panza, L, Riva, S & Bellini, T 2005, Light scattered by model phantom bacteria reveals molecular interactions at their surface,University of Milano Press,Segrate.
Gordeeva, V, E 1992, “COMPASS program – an original semi-empirical approach to computer-assisted synthesis”, Journal of Tetrahedron Compounds, vol. 48 no. 7, pp. 3789.
Hansjuergen, S, Christoph, G, J 1956, The Extension of the Pinner Synthesis of Monohydroxy-s-triazines to the Aliphatic Series. 2,4-Dimethyl-s-triazine1, Journal of Multicomponent reactions vol. 78 no. 11, pp 2447–2451.
Ivar, U 2001, Recent progress in the chemistry of multicomponent reactions, Pure Applied Chemical, vol. 73 no. 1, pp. 187-191.
Jackson, P & Haddad, P, R 1990, Ion chromatography: principles and applications, Journal of Chromatography Library, vol. 58, pp.74.
Jiang, B, Hao, W, J, Wang, X, Shi, F & Tu, S, J 2009, Diversity-oriented synthesis of Krohnke pyridines. Journal of Combinatorial Chemistry, vol.11, no. 5, pp. 846–850.
Jiang, B, Li, C, Shi, F, Tu, S, J, Kaur, P, Wever, W & Li, G 2010, Four-component domino reaction providing an easy access to multifunctionalized tricyclo[6.2.2.01,6]dodecane derivatives. Journal of Organic Chemistry, vol. 75, no. 9, pp. 2962-2965.
Kaim, E, Gizolme, M, Grimaud, L & Oble, J 2006, Direct Access to Heterocyclic Scaffolds by New Multicomponent Ugi-Smiles Couplings, Journal of Organic Chemestry, vol. 72 no. 2, pp 544 – 547.
Kenneth, S 2009, “Sonochemistry.” Access Science. Web.
Khersonsky, S, M, Jung, D, W, Kang, T, W, Walsh, D, P, Moon, H, S, Jo, H, Jacobson, E, M, Shetty, V, Neubert,T, A & Chang, Y, T 2003, Journal of American Chemical Society, vol. 125.
Kumar, A 2008, ‘Efficient Synthesis of Hantzsch Esters and Polyhydro- quinoline Derivatives in Aqueous Micelles’, Journal of Organic Chemestry, vol. 3, pp.883.
Ma, Z, Xiang, Z, Luo, T, Lu, K, Xu, Z, Chen, J & Yang, J, Z 2006, Synthesis of Functionalized Quinolines via Ugi and Pd-Catalyzed Intramolecular Arylation Reactins Journal on combinatorial Chemistry,vol.8 no.5, pp. 696 – 704.
Mardare, A 2008, “Combinatorial solid state materials science and technology” Journal of Science and Technology, vol. 10 no.87, pp. 33-46.
Miyaura, N & Suzuki, A 1979. “Palladium-Catalyzed Cross-Coupling Reactions of Organoboron Compounds”, Plenum Press, New York.
Miyaura, N, Yamada, K & Suzuki, A 1979, “A new stereospecific cross-coupling by the palladium-catalyzed reaction of 1-alkenylboranes with 1-alkenyl or 1-alkynyl halides”, Journal of Organic Chemestry, vol. 6, pp. 543-548.
Moreira, P, I, Zhu, X, Nunomura, A, Smith, M, A & Perry, G 2006, Therapeutic Options in Alzheimer’s disease. Expert Rev. Neurother. University of Paris Publications, Paris.
Muarya, A, R 2008, ‘Organocatalyzed three-component domino synthesis of 1,4-dihydropyridines under solvent free conditions Tetrahedron’, Journal of Organic Chemestry, vol. 64, pp. 3477-3482.
Newman, D & Cragg, G 2007, “Natural Products as Sources of New Drugs over the Last 25 Years”, Journal of Natural products, vol. 7 no. 461, 336-390.
Nicholas E, L & Smith, J, R 2006, “Real-Time Monitoring of Microwave-Promoted Suzuki Coupling Reactions Using in Situ Raman Spectroscopy.” Organic Letters, vol. 20, no. 8, pp. 4589-591. Web.
Nicholas, E, L & Marco, M 2003 “Rapid and Amenable Suzuki Coupling Reaction in Water Using Microwave and Conventional Heating.” The Journal of Organic Chemistry, vol. 68, no.3, pp. 888-92. Web.
Pirrung, M 2005, Microwave accelerated reactions, Wayne State University Press, Detroit, Michigan.
Pirrung, M, C & Sarma, K, D 2004, “Multicomponent Reactions Are Accelerated in Water”. Journal of the American Chemical Society, vol. 126 no.2, pp. 444–445.
Price, G, L 2007, TU Chemical Engineering Zeolite Page. Web.
Rossen, K, Pye, P, J, DiMichele, L, M, Volante, R, P & Reider, P, J 1998, “An efficient asymmetric hydrogenation approach to the synthesis of the Crixivan piperazine intermediate”,Journal of Tetrahedron Compounds, vol.39, pp. 6823–6825.
Sambasivarao, K, Kakali, L & Dhurke, K 2002, Recent applications of the Suzuki–Miyaura cross-coupling reaction in organic synthesis, Tetrahedral Journal, vol. 58 no. 48, pp. 9633-9695.
Short, K, M & Mjalli, A, M, M 1997, “A solid-phase combinatorial method for the synthesis of novel 5- and 6-membered ring lactams”, Journal of Tetrahedron Letters, vol.38 no.18, pp. 359–362.
Simons, R 1890, Saxton Organic Syntheses, Journal of Multicomponent reactions, Vol. 4, no 9, p.78.
Sun, H, Brooks, M, M, Panza, L, Matto, P, Compostella, F, Remmel, N, Klingenstein, R, Sandhoff, K, Fluharty, C, Fluharty, A & Faull, K, F 2000, A novel mass spectrometric assay for the cerebroside sulfate activator protein (saposin B) and arylsulfatase,East African Publishers, Uganda.
Tempest, P, A 2005, “Recent advances in heterocycle generation using the efficient Ugi multiple-component condensation reaction”. Journal of Current Opinion in Drug Discovery & Development,vol, 8 no.6, pp. 776–788.
Ugi, I & Heck, S, 2001, “The multicomponent reactions and their libraries for natural and preparative chemistry”, Journal of Combinatorial Chemistry & High Throughput Screening,vol. 4 no.1, pp. 1–34.
Ugi, I, 1962, “The α-Addition of Immonium Ions and Anions to Isonitriles Accompanied by Secondary Reactions”, Journal of Chemical Compounds, vol. 62, pp. 67-68.
Xiang, D 1995, “A Combinatorial Approach to Materials Discovery”, Journal of Molecular Science, vol. 268. pp.1738.
Xiang, Z, Luo, T, Cui, J, Shi, X, Fathi, R, Chen, J & Yang, Z 2004, “Novel Pd-II-mediated cascade carboxylative annulation to construct benzo[b]furan-3-carboxylic acids” Journal of Organic Chemestry, vol.6 no.24, pp. 345-346.
Yongxiang, L, Lu, K, Dai, M, Wu, W, Chen, J, Quan, J & Yang, Z 2007 “An Efficient One-Pot Asymmetric Synthesis of Biaryl Compounds.” Organic Letters , pp. 805-808. Web.
Zhang, J, Jacobson, A, Rusche, J, R & Herlihy, W 1999, “Unique Structures Generated by Ugi 3CC Reactions Using Bifunctional Starting Materials Containing Aldehyde and Carboxylic Acid”, Journal of Organic Chemistry, vol 64 no.4, pp. 1074.